新闻动态
...novel object recognition in the hypergravity-exposed rats...
2024-04-27
Impairment of synaptic plasticity and novel object recognition in the hypergravity-exposed rats AbstractThe gravity is necessary for living organisms to operate various biological events including hippocampus-related functions of learning and memory. Until now, it remains inconclusive how altered gravity is associated with hippocampal functions. It is mainly due to the difficulties in generating an animal model experiencing altered gravity. Here, we demonstrate the effects of hypergravity on hippocampus-related functions using an animal behavior and electrophysiology with our hypergravity animal model. The hypergravity (4G, 4聽weeks) group showed impaired synaptic efficacy and long-term potentiation in CA1 neurons of the hippocampus along with聽the poor performance of a novel object recognition task. Our studies suggest that altered gravity affects hippocampus-related cognitive functions, presumably through structural and functional adaptation to various conditions of gravity shift. IntroductionGravity shift renders living organisms to change their physiological properties and become evolved and adapted for stabilization to the current environment. Gravitational change such as microgravity (MG) and hypergravity (HG) is the most influential stressor to terrestrial and aquatic creatures from Animalia to Plantae1,2,3,4,5,6,7. As for human, astronauts who experience both MG (in outer space) and HG (in launch and re-entry)8 suffer from various adverse symptoms9,10,11. Gravity shift from the space to Earth can be considered much larger HG to astronauts, and they are likely to spend聽a long time under intensive HG. Also, the level of HG used for the聽study on enhancement of drug effect is massive12,13,14,15. Under this condition, the聽central nervous system (CNS) is susceptible to gravity in the context of movement control, sensory integration, locomotion, balance, circadian rhythm, and hormone release16,17,18,19,20,21,22,23,24,25,26,27,28,29.Hippocampus is the essential part of CNS in light of learning and memory30,31,32,33,34,35,36,37,38. The effect of gravity on the聽hippocampus is investigated in various methods of behavioral, molecular, and electrophysiological aspects. MG causes the impaired discrimination of a new spatial arrangement39,40 and alteration in neural proteome39,41, gene expression42 and cytomorphometry43. Interestingly, short-term exposure of HG (4G, 48聽h) results in enhanced LTP on CA144 while long-term exposure of HG (4G, 3聽weeks) impairs spatial learning performance45. Various effects of gravity on hippocampus-related functions from genetic to molecular, behavioral, and electrophysiological levels are summarized in Table 139,40,41,42,43,44,45,46,47,48,49,50,51,52,53,54,55, based on the duration and induction method of MG and HG. However, synaptic mechanism and behavioral consequence of HG on hippocampal functions still remain undetermined.Table 1 Previous studies summarizing the聽physiological effects of altered gravity on hippocampus-related functions from genetic to molecular, behavioral, and electrophysiological levels.Full size tableThe aim of current study is to reveal a certain relationship between gravity and the hippocampal synaptic mechanisms underlying a cognitive function. For this, we employed a centrifugal system for gravity conditioning and electrophysiology (EPG) for observing synaptic responses of hippocampal CA1 neurons along with the behavior test of novel object recognition (NOR). Our results show that the ability聽to discriminate a novel object from the familiar one is impaired in the long-term exposure of HG (4聽weeks) but not in the short-term exposure of HG (1聽day). Furthermore, postsynaptic responses were reduced under long-term HG, largely due to the impairment of 伪-amino-3-hydroxy-5-methyl-4-isoxazolepropionic acid receptors (AMPARs) and N-methyl-d-aspartate receptors (NMDARs). Here, we demonstrate that the long-term HG impairs hippocampus-related synaptic functions, therein suggesting the cellular mechanism of a HG-induced cognitive deficit and a聽therapeutic strategy.Experimental proceduresAnimalsAbout 11聽weeks old male Sprague鈥揇awley (SD) rats were used for electrophysiology and animal behavior test. All animal handling procedures were approved by the Institutional Animal Care and Use Committee of Inha University (INHA 180105-533) and Incheon National University (INU-ANIM-2017-08), and all experiments were performed in accordance with relevant guidelines and regulations.Hypergravity exposureSD rats were conditioned by HG (4G) induced in a gravitational force simulator with two horizontal rotatory arms (50聽cm long each). When the arms were rotated, centrifugal force was delivered to the animal cage locating at the end of arms. When the arms rotated at a speed of 65聽rpm, rats in the cage were exposed to 4G. A high-resolution camera inside the cage was used to observe whether rats were able to move freely and access food and water. The rats were exposed to HG for 23聽h and took an hour rest under normal gravity (1G). The conditioning process was repeated for 1聽day (HG1day)聽or 4聽weeks (HG4weeks) (Fig.聽1a). The behavior test and EPG were conducted in 24聽h right after the rats were released from HG (Fig.聽1b).Figure 1Conditioning and experiment procedure. (a) Schematic diagram showing HG exposure processes. It shows 1-day conditioning process that comprises 23聽h HG exposure followed by 1-h rest period. It is repeated for 4聽weeks. (b) Schematic diagram for overall time schedule: HG exposure (1聽day or 4聽weeks), behavior test (7聽h), and EPG (21聽h).Full size imageBehavior test of a novel object recognitionNOR test comprised two phases. Each phase with 10聽min duration was separated by a 6-h inter-phase interval. During the first phase of the familiarization period, the rat was presented in a pair of identical, familiar objects (F1, F2) in a white acrylic open field box (60聽cm width鈥壝椻€?0聽cm length鈥壝椻€?0聽cm height) to be habituated to environmental factors including the place of objects. The two objects were placed in a diagonal position about 5聽cm away from the white wall (Fig.聽2a). The exploratory聽movement of the rat during the phase was recorded with a video camera installed at the top of the apparatus. In completing the first phase, the rat and familiar objects (F1, F2) were removed from the apparatus for 6聽h. In the second phase, the test phase, the rat explored a third copy of the familiar object (F3) and a novel object (N) in the apparatus. Rats used to explore the novel object (N) more than the familiar one (F3). To eliminate a certain variation by emotional instability, rats with excessive freezing behavior more than 60% ( 鈥?60 s) out of聽the whole period of test phase (10聽mins) were excluded from analysis (Exclusion : 1G鈥? out of 10 rats; HG1day, HG4weeks鈥攅ach 1 out of 5 rats). Object exploration was defined when the nose of the rats directed towards the object at a distance below 2聽cm and measured by the聽discrimination index which indicates聽the difference of time spent between聽a novel (TN) and familiar object (TF3). It was calculated with the total amount of time spent with both objects in the test phase [Discrimination Index鈥?鈥?TN鈥擳F3)/(TN鈥?鈥塗F3)].Figure 2Novel object recognition (NOR) test with 1G and HG rats. (a) The scheme of arena and the position of objects. (b) Representative traces of 1G, HG1day and HG4weeks for each phase (familiarization and test). (c) Exploration times for each object during the聽familiarization phase. (d) HG4weeks group had no preference for聽both F3 and N objects during the聽test phase. (e) Discrimination indexes are plotted as a function of the 1G and HG groups. HG4weeks impairs the discrimination of novel objects. (f) Total exploration time does not show any differences between the groups. *鈮?0.05 *** 鈥?.001, n.s not significant.Full size imageBrain slice preparationAge matched conditioned (HG group) and unconditioned (1G group) rats were deeply anesthetized with 2% isoflurane. Motionless rats were decapitated by a guillotine, and the brain was isolated quickly. The isolated brain was transferred to the ice-cold dissection buffer containing the following ingredients (in mM): 25 glucose, 75 sucrose, 87 NaCl, 2.5 KCl, 1.3 NaH2PO4, 25 NaHCO3, 7.0 MgCl2, and 0.5 CaCl2 bubbled with a mixture of 5% CO2 and 95% O2. The hippocampus including Schaffer collaterals (SC) was isolated from the whole brain and transversely sectioned into 400聽渭m thick slices using Leica VT1200S vibratome (Leica Biosystems, Wetzlar, Germany) filled with the same buffer. The slices were incubated for 12聽min at 32聽掳C and聽recovered for 1聽h at room temperature,聽submerged in the聽artificial cerebral spinal fluid (aCSF) containing the following ingredients (in mM): 25 glucose, 125 NaCl, 2.5 KCl, 1.3 NaH2PO4, 25 NaHCO3, 1.0 MgCl2, and 2.0 CaCl2 bubbled with a mixture of 5% CO2 and 95% O2.In vitro field recordingSlices were transferred to a recording chamber where aCSF flowed (31鈥壜扁€?.5聽掳C; 1鈥?聽ml/min). A slice harp anchored the slices in order to stabilize聽the recording position. A bipolar stimulating electrode was placed on the SC to evoke field excitatory postsynaptic potential (fEPSP) which were recorded on the stratum radiatum (SR) of CA1 by glass electrodes filled with aCSF. All responses were acquired using Axon Digidata 1550B 8-Channel Digitizer (Molecular Devices, San Jose, CA) and amplified using MultiClamp 700B Microelectrode amplifier (Molecular Devices, San Jose, CA, USA). The maximum slope of fEPSPs was identified in the establishment of input鈥搊utput (I/O) relationship. Half maximal fEPSPs were used for high frequency stimulation (HFS: 100聽Hz, 1聽s) for LTP induction.Data analysisAll electrophysiological data were presented numerically using Axon pCLAMP11 Electrophysiology Data Acquisition and Analysis Software (Molecular Devices, San Jose, CA). The difference in behavior assessment was measured by One-way analysis of variance (ANOVA) of the Bonferroni post-hoc test. Two-way ANOVA was used to assess statistical significance for the differences between HG and 1G groups in EPG. Every statistical process was performed on SPSS Statics 25 (IBM, Armonk, NY). At least p鈥?lt;鈥?.05 was interpreted statistically significant (*p鈥?lt;鈥?.05, **p鈥?lt;鈥?.01, ***p鈥?lt;鈥?.001). All Graphs were prepared by GraphPad Prism 7 (GraphPad Software Inc., La Jolla, CA, USA) and final arrangement and labeling were carried out using Adobe Illustrator CC 2019 (Adobe Inc., San Jose, CA, USA). All data are presented in mean鈥壜扁€塻tandard error of the mean (SEM). N indicates the number of animals in the NOR test and slices in EPG.ResultImpaired NOR in rats under HGWe wondered whether the gravity affects memory-related behavior according to an聽exposure time of HG. The NOR task was conducted with rats under normal gravity, HG1day, and HG4weeks in open field box (Fig.聽2a,b). Rats showed similar preference for each object regardless of HG in the聽familiarization phase (Fig.聽2c; 1G鈥擣1: 31.50鈥壜扁€?.64, F2: 37.58鈥壜扁€?.40, p鈥?鈥?.590, HG1day鈥擣1: 31.32鈥壜扁€?.79, F2: 30.40鈥壜扁€?.73, p鈥?鈥?.906, HG4weeks鈥擣1: 59.28鈥壜扁€?0.82, F2: 52.37鈥壜扁€?0.46, p鈥?鈥?.662). 1G and HG1day had a preference for novel objects in聽the test phase but not HG4weeks rats (Fig.聽2d; 1G鈥擣3: 27.20鈥壜扁€?.12, N: 51.28鈥壜扁€?.06, p鈥?lt;鈥?.001, HG1day鈥擣3: 22.46鈥壜扁€?.80, N: 47.38鈥壜扁€?0.14, p鈥?鈥?.052, HG4weeks鈥?/sub>F3: 45.19鈥壜扁€?.56, N: 43.09鈥壜扁€?.59, p鈥?鈥?.831). Discriminating competence differed between groups (Fig.聽2e; 1G鈥?.31鈥壜扁€?.03, n鈥?鈥? rats, HG1day鈥?.33鈥壜扁€?.06, n鈥?鈥? rats, HG4weeks鈥?.02鈥壜扁€?.04, n鈥?鈥? rats, F2,12鈥?鈥?8.224, p鈥?lt;鈥?.001). The 1G and HG1day groups preferred a novel object whereas the聽HG4weeks group聽showed the聽similar preference toward both objects (posttest: 1G vs. HG1day鈥?i>p鈥?鈥?.000, 1G vs. HG4weeks鈥?i>p鈥?lt;鈥?.001, HG1day vs. HG4weeks鈥?i>p鈥?鈥?.001). To determine if HG influences motivation and locomotion for rats to explore, total exploration time was measured (Fig.聽2f; 1G鈥?8.47鈥壜扁€?.91, n鈥?鈥? rats, HG1day鈥?9.84鈥壜扁€?1.74, n鈥?鈥? rats, HG4weeks鈥?8.28鈥壜扁€?2.78, n鈥?鈥? rats, F2,12鈥?鈥?.727, p鈥?鈥?.503), showing no significant difference between all groups. It indicates that HG4weeks impairs the ability聽to discriminate a novel object from聽the familiar one.Reduced postsynaptic, but not presynaptic transmission under HGIn order to examine whether HG affects synaptic events in the hippocampal CA1 network, we tested the聽synaptic transmission of CA1 pyramidal cells in response to SC stimulation. The fiber volley (FV), the聽indicator of Ca2+ influx into the presynaptic axon terminal, was considered as the input while the slope of postsynaptic聽fEPSPs, mostly AMPARs-mediated responses, was taken as the output. As predicted, the slope of fEPSPs increased as the amplitude of FVs increased in both groups (Fig.聽3a,b; 1G鈥?.2聽mV: 0.15鈥壜扁€?.02, n鈥?鈥?7 slices, 0.4聽mV: 0.38鈥壜扁€?.03, n鈥?鈥?0 slices, 0.6聽mV: 0.66鈥壜扁€?.14, n鈥?鈥?4 slices; HG鈥?.2聽mV: 0.08鈥壜扁€?.01, n鈥?鈥?0 slices, 0.4聽mV: 0.23鈥壜扁€?.06, n鈥?鈥?2 slices, 0.6聽mV: 0.34鈥壜扁€?.11, n鈥?鈥?0 slices). However, the聽significant deficit of synaptic transmission was observed in the HG group when it is compared to that in the 1G group (F1,127鈥?鈥?4.747, p鈥?lt;鈥?.001). A post-hoc Student鈥檚 t tests showed the significant difference in output at 0.2 and 0.4聽mV (t0.2聽mV鈥?鈥?.091, p0.2聽mV鈥?鈥?.004, t0.4聽mV鈥?鈥?.217, p0.4聽mV鈥?鈥?.033, t0.6聽mV鈥?鈥?.766, p0.6聽mV鈥?鈥?.091). Next, to test whether the response difference is attributed to altered function of NMDARs, NMDAR response as the function of FVs was measured in the presence of 10聽渭M NBQX, an AMPAR antagonist, and 0聽mM MgCl2,聽an NMDAR-enhancing chemical. We found that the HG group had the聽significant reduction in NMDAR response when compared with that of the 1G group (Fig.聽3c; 1G鈥?.2聽mV: 0.32鈥壜扁€?.04, n鈥?鈥?0 slices, 0.4聽mV: 0.60鈥壜扁€?.10, n鈥?鈥?2 slices, 0.6聽mV: 1.19鈥壜扁€?.04, n鈥?鈥? slices; HG鈥?.2聽mV: 0.18鈥壜扁€?.02, n鈥?鈥? slices, 0.4聽mV: 0.35鈥壜扁€?.05, n鈥?鈥?0 slices, 0.6聽mV: 0.57鈥壜扁€?.10, n鈥?鈥?1 slices; F1,46鈥?鈥?2.989, p鈥?lt;鈥?.001). A post-hoc Student鈥檚 t tests showed the significant difference at all intensities of FVs (t0.2聽mV鈥?鈥?.399, p0.2聽mV鈥?鈥?.032, t0.4聽mV鈥?鈥?.704, p0.4聽mV鈥?鈥?.001, t0.6聽mV鈥?鈥?.779, p0.6聽mV鈥?lt;鈥?.001). Also, the amplitude of FVs was measured over various intensities in order to test whether HG affects presynaptic transmission. In the HG group, there was no deficit of FV responses over the increasing intensities. (Fig.聽3d; 1G鈥?0渭A: 0.08鈥壜扁€?.01, n鈥?鈥? slices, 25 渭A: 0.11鈥壜扁€?.03, n鈥?鈥? slices, 50渭A: 0.16鈥壜扁€?.04, n鈥?鈥? slices, 75 渭A: 0.28鈥壜扁€?.04, n鈥?鈥? slices, 100 渭A: 0.31鈥壜扁€?.05, n鈥?鈥? slices, 125 渭A: 0.35鈥壜扁€?.05, n鈥?鈥? slices, 150 渭A: 0.40鈥壜扁€?.07, n鈥?鈥? slices, 175 渭A: 0.45鈥壜扁€?.07, n鈥?鈥? slices, 200 渭A: 0.49鈥壜扁€?.07, n鈥?鈥? slices; HG鈥?0 渭A: 0.05鈥壜扁€?.02, n鈥?鈥? slices, 25 渭A: 0.13鈥壜扁€?.03, n鈥?鈥? slices, 50 渭A: 0.20鈥壜扁€?.07, n鈥?鈥? slices, 75 渭A: 0.20鈥壜扁€?.06, n鈥?鈥? slices, 100 渭A: 0.28鈥壜扁€?.07, n鈥?鈥? slices, 125 渭A: 0.29鈥壜扁€?.10, n鈥?鈥? slices, 150 渭A: 0.36鈥壜扁€?.11, n鈥?鈥? slices, 175 渭A: 0.40鈥壜扁€?.11, n鈥?鈥? slices, 200 渭A: 0.42鈥壜扁€?.13, n鈥?鈥? slices; F1,114鈥?鈥?.152, p鈥?鈥?.285). Our data demonstrate that HG likely damages the postsynaptic function in the SC-CA1 synapse.Figure 3Impaired postsynaptic AMPAR / NMDAR function, but not presynaptic transmission, in聽the hippocampal CA1 network. (a) Representative traces of FVs and fEPSPs at 0.2聽mV (from the聽arrow in b). (b) The slope of fEPSPs as the function of the increasing amplitudes of FVs. HG reduces fEPSPs at 0.2 and 0.4聽mV FVs. (c) Pharmacologically isolated NMDAR mediated responses over the increasing FVs in the presence of 10聽渭M NBQX and 0聽mM MgCl2. HG reduces the amplitude of NMDAR responses at all FVs. (d) The amplitude of FVs over various stimulus intensities does not show any difference between 1Gl and HG groups.Full size imageAltered LTP in HGTo test whether HG has an effect on synaptic plasticity, we examine short-term and long-term synaptic plasticity in both the 1G and HG groups. When SC was activated by the paired pulse with various intervals, differential paired pulse facilitation (PPF) was observed as the slope of the second fEPSPs (P2) over the first fEPSPs (P1) in both groups (Fig.聽4a). There was no statistical聽significance between the two groups (1G鈥?0聽ms: 1.98鈥壜扁€?.23, n鈥?鈥? slices, 100聽ms: 1.77鈥壜扁€?.17, n鈥?鈥? slices, 250聽ms: 1.24鈥壜扁€?.07, n鈥?鈥? slices, 500聽ms: 1.10鈥壜扁€?.07, n鈥?鈥? slices, HG鈥?0聽ms: 1.95鈥壜扁€?.20, n鈥?鈥?4 slices, 100聽ms: 1.89鈥壜扁€?.20, n鈥?鈥?6 slices, 250聽ms: 1.22鈥壜扁€?.06, n鈥?鈥?3 slices, 500聽ms: 1.09鈥壜扁€?.06, n鈥?鈥?5 slices; F1,86鈥?鈥?.011, p鈥?鈥?.915). It is remarkable to observe that LTP was diminished in the HG group (Fig.聽4b; 1G鈥攂aseline: 101.28鈥壜扁€?.15, post HFS: 158.71鈥壜扁€?.91, n鈥?鈥?1 slices; HG鈥攂aseline: 102.27鈥壜扁€?.13, post HFS: 110.65鈥壜扁€?.87, n鈥?鈥?3 slices; F1,220鈥?鈥?3.070, p鈥?鈥?.001). Our results suggest that聽the gravity shift plays a critical role in the long-term synaptic plasticity.Figure 4Impaired long-term synaptic plasticity under HG. (a) PPF was聽calculated by the聽P2/P1 ratio over various inter-stimulus intervals. P2/P1 of HG groups is not significantly different from that of 1G groups. (b) HG impairs LTP induced by HFS. Representative traces of both baselines (gray line) and LTP after HFS (black and red lines) are indicated, showing a聽negligible increase of LTP under HG.Full size imageDiscussionIt has been well studied that the vestibular system is greatly affected by altered gravity21,22,56,57,58. The previous study implied that聽the vestibular organ was the main area influenced by HG in the brain48. Notably, the聽hippocampus, especially CA1, had electrophysiological and anatomical connections with the vestibular system59,60,61,62,63,64,65,66. For example, lesioned hippocampus aggravated HG-induced motion sickness53. The fact that HG has adverse effects on the brain triggered us to investigate a hippocampal function after聽the HG conditioning. Our current findings are as follows (1) HG4weeks causes the behavioral deficit in the NOR test; (2) HG4weeks impairs AMPARs/NMDARs-mediated synaptic transmission; (3) HG4weeks group shows abnormal postsynaptic (but normal presynaptic) responses; and (4) HG4weeks alters LTP.Differential effects of HG on pre- and post-synaptic neuronsNeurotransmitter release is determined by the incidence and pattern of action potentials, depolarization of nerve terminals, and release probability of vesicle machinery67,68,69,70. The previous study investigating the聽synaptosome in cerebral hemispheres showed that HG alters neurotransmitter release by modulation of neurotransmitter reuptake, indicating a role of HG in a presynaptic mechanism71. However, in our study, there was no significant difference of presynaptic FV amplitude over stimulation intensities and PPF representing presynaptic Ca2+ influx and neurotransmitter release-probability, respectively. It demonstrates that HG does not alter the presynaptic activity at least in CA3鈥揅A1 network. Instead, the strong reliance on postsynaptic AMPAR/NMDAR responses under HG condition depicts a postsynaptic mechanism. This finding is consistent with our early study that HG causes聽the impaired function of postsynaptic AMPAR and metabotropic glutamate receptors (mGluRs) subtype 1 in聽the cerebellum72.A role of HG in cognitive behaviors and plasticityPrevious studies showed that HG causes various physiological changes, such as vestibular function73, signaling pathway in muscles74, and bone formation75 which can lead to altered behaviors. As expected, our research group also observed that HG causes an abnormality of cerebellum-dependent motor coordination72. Now, our view is expanded to investigate a role of HG in cognitive behaviors with a memory test. HG-driven poor performance in NOR may be affected by a defect of memory function because NOR is dominantly dependent on the聽hippocampus76,77,78,79,80. Our result suggests that HG directly triggers the dysfunction of the hippocampus-dependent cognitive behavior. Given our and other results, HG could accompany multiple, parallel processing of various physiological systems such as HG to vestibular/motor behaviors and HG to cognitive behaviors.It is previously well known that NMDARs are deeply involved in neural plasticity and often behaviors32,36,81,82,83. A previous study described an increased LTP under short-term exposure of 4G (48聽h)44. Meanwhile, we observed that the long-term, but not short-term, exposure of 4G (4聽weeks) impairs a cognitive behavior followed by LTP deficit. Prior studies (various gravity levels, 3聽weeks) revealed that HG-induced abnormality in various behaviors was sustained even after 15聽days from centrifugation45. In our experimental condition, it seems to be worth testing how long the HG effect lasts.Therapeutic strategy for HGThis study provides the scientific aspect of physiological effects by HG on hippocampus. Long-term gravity shift can cause the impairment of electrophysiological property in the聽hippocampus and聽the behavior in聽the NOR task, and it could be due to a defect of postsynaptic receptors. Therefore, HG-induced impairment may have聽the potential to be rescued by restoring the function of postsynaptic receptors. We have previously proposed transient potassium channels as a therapeutic target for various brain disorders. It is because the transient potassium channels are electrically counteracting channels to NMDARs and have clinical benefits of minimal interference in a聽normal synaptic transmission which can be impaired under the pharmacological modulation of NMDARs84,85. A pharmacological approach to enhance an NMDAR function can be further investigated under the condition of altered gravity. References1.Goldermann, M. Hanke, W. Ion channel are sensitive to gravity changes. Microgravit. Sci. Technol. 13, 35鈥?8 (2001).ADS聽 CAS聽 Article聽Google Scholar聽 2.Ilyin, E. A. Oganov, V. S. Microgravity and musculoskeletal system of mammals. Adv. Sp. Res. 9, 11鈥?9 (1989).ADS聽 CAS聽 Article聽Google Scholar聽 3.Gustave Dit Duflo, S., Gestreau, C. Lacour, M. Fos expression in the rat brain after exposure to gravito-inertial force changes. Brain Res. 861, 333鈥?44 (2000).CAS聽 PubMed聽 Article聽Google Scholar聽 4.Cox, J. F. et al. Influence of microgravity on astronauts鈥?sympathetic and vagal responses to Valsalva鈥檚 manoeuvre. J. Physiol. 538, 309鈥?20. https://doi.org/10.1113/jphysiol.2001.012574 (2002).CAS聽 Article聽 PubMed聽 PubMed Central聽Google Scholar聽 5.Gharib, C. Custaud, M. A. Orthostatic tolerance after spaceflight or simulated weightlessness by head-down bed-rest. Bull. Acad. Natl. Med. 186, 733鈥?46 (2002).PubMed聽Google Scholar聽 6.Chabbert, C., Brugeaud, A., Lennan, G., Lehouelleur, J. Sans, A. Electrophysiological properties of the utricular primary transducer are modified during development under hypergravity. Eur. J. Neurosci. 17, 2497鈥?500 (2003).PubMed聽 Article聽Google Scholar聽 7.Rahmann, H., Slenzka, K., Kortje, K. H. Hilbig, R. Synaptic plasticity and gravity: Ultrastructural, biochemical and physico-chemical fundamentals. Adv. Sp. Res. 12, 63鈥?2 (1992).ADS聽 CAS聽 Article聽Google Scholar聽 8.Pompeiano, O. et al. Immediate early gene expression in the vestibular nuclei and related vegetative areas in rats during space flight. Acta Otolaryngol. Suppl. 545, 120鈥?26 (2001).CAS聽 PubMed聽 Article聽Google Scholar聽 9.Heer, M. Paloski, W. H. Space motion sickness: Incidence, etiology, and countermeasures. Auton. Neurosci. 129, 77鈥?9 (2006).PubMed聽 Article聽Google Scholar聽 10.Van Ombergen, A. et al. Brain tissue-volume changes in cosmonauts. N. Engl. J. Med. 379, 1678鈥?680. https://doi.org/10.1056/NEJMc1809011 (2018).Article聽 PubMed聽Google Scholar聽 11.Roberts, D. R. et al. Effects of spaceflight on astronaut brain structure as indicated on MRI. N. Engl. J. Med. 377, 1746鈥?753. https://doi.org/10.1056/NEJMoa1705129 (2017).Article聽 PubMed聽Google Scholar聽 12.Kim, D., Kim, Y. H. Kwon, S. Enhanced nasal drug delivery efficiency by increasing mechanical loading using hypergravity. Sci. Rep. 8, 168 (2018).ADS聽 PubMed聽 PubMed Central聽 Article聽 CAS聽Google Scholar聽 13.Jang, T. Y., Jung, A.-Y., Kwon, S. Kim, Y. H. Hypergravity enhances the therapeutic effect of dexamethasone in allergic asthma and rhinitis animal model. PLoS聽One 13, e0197594 (2018).PubMed聽 PubMed Central聽 Article聽 CAS聽Google Scholar聽 14.Genchi, G. G. et al. Hypergravity as a tool for cell stimulation: Implications in biomedicine. Front. Astronomy Sp. Sci. 3, 26 (2016).ADS聽Google Scholar聽 15.Cogoli, A. The effect of hypogravity and hypergravity on cells of the immune system. J. Leukoc. Biol. 54, 259鈥?68 (1993).CAS聽 PubMed聽 Article聽Google Scholar聽 16.Rosenzweig, M. R., Bennett, E. L. Diamond, M. C. Brain changes in response to experience. (1972).17.Fuller, C. A. The effects of gravity on the circadian timing system. J. Gravit. Physiol. 1, P1-4 (1994).CAS聽 PubMed聽Google Scholar聽 18.Daunton, N. G., Tang, F., Corcoran, M. L., Fox, R. A. Man, S. Y. Chronic exposure to hypergravity affects thyrotropin-releasing hormone levels in rat brainstem and cerebellum. Biol. Signals Recept. 7, 337鈥?44. https://doi.org/10.1159/000014557 (1998).CAS聽 Article聽 PubMed聽Google Scholar聽 19.Maru拧i膷, U., Meeusen, R., Pi拧ot, R. Kavcic, V. The brain in micro-and hypergravity: The effects of changing gravity on the brain electrocortical activity. Eur. J. Sport Sci. 14, 813鈥?22 (2014).PubMed聽 Article聽Google Scholar聽 20.Jang, T. Y., Jung, A. Y. Kim, Y. H. Hormetic effect of chronic hypergravity in a mouse model of allergic asthma and rhinitis. Sci. Rep. 6, 27260. https://doi.org/10.1038/srep27260 (2016).ADS聽 CAS聽 Article聽 PubMed聽 PubMed Central聽Google Scholar聽 21.Gaboyard, S., Sans, A. Lehouelleur, J. Differential impact of hypergravity on maturating innervation in vestibular epithelia during rat development. Brain Res. Dev. Brain Res. 143, 15鈥?3 (2003).CAS聽 PubMed聽 Article聽Google Scholar聽 22.Bruce, L. L. Adaptations of the vestibular system to short and long-term exposures to altered gravity. Adv. Sp. Res. 32, 1533鈥?539. https://doi.org/10.1016/S0273-1177(03)90392-9 (2003).ADS聽 CAS聽 Article聽Google Scholar聽 23.Kramer, A., Gollhofer, A. Ritzmann, R. Acute exposure to microgravity does not influence the H-reflex with or without whole body vibration and does not cause vibration-specific changes in muscular activity. J. Electromyogr. Kinesiol. 23, 872鈥?78 (2013).PubMed聽 Article聽Google Scholar聽 24.Ritzmann, R., Freyler, K., Weltin, E., Krause, A. Gollhofer, A. Load dependency of postural control-kinematic and neuromuscular changes in response to over and under load conditions. PLoS聽One 10, e0128400 (2015).PubMed聽 PubMed Central聽 Article聽 CAS聽Google Scholar聽 25.Schaffhauser, D. F. et al. Microfluidic platform for electrophysiological studies on Xenopus laevis oocytes under varying gravity levels. Lab Chip 11, 3471鈥?478 (2011).CAS聽 PubMed聽 Article聽Google Scholar聽 26.Sato, T., Miyoshi, T., Nakazawa, K., Yano, H. Takeoka, H. Reflex response changes during hyper and microgravity. J. Gravit. Physiol. 8, P97-99 (2001).CAS聽 PubMed聽Google Scholar聽 27.Ohira, Y., Nomura, T., Kawano, F., Soduh, M. Ishihara, A. Responses of Hoffman-reflex in human soleus to gravity and/or fluid shift. J. Gravit. Physiol. 9, P129-130 (2002).PubMed聽Google Scholar聽 28.Miyoshi, T. et al. Somatosensory graviception inhibits soleus H-reflex during erect posture in humans as revealed by parabolic flight experiment. Exp. Brain Res. 150, 109鈥?13 (2003).PubMed聽 Article聽Google Scholar聽 29.Crone, C. et al. Sensitivity of monosynaptic test reflexes to facilitation and inhibition as a function of the test reflex size: A study in man and the cat. Exp. Brain Res. 81, 35鈥?5 (1990).CAS聽 PubMed聽 Article聽Google Scholar聽 30.Jarrard, L. E. On the role of the hippocampus in learning and memory in the rat. Behav. Neural Biol. 60, 9鈥?6. https://doi.org/10.1016/0163-1047(93)90664-4 (1993).CAS聽 Article聽 PubMed聽Google Scholar聽 31.Broadbent, N. J., Squire, L. R. Clark, R. E. Spatial memory, recognition memory, and the hippocampus. Proc. Natl. Acad. Sci. USA 101, 14515鈥?4520. https://doi.org/10.1073/pnas.0406344101 (2004).ADS聽 CAS聽 Article聽 PubMed聽Google Scholar聽 32.Yang, S. et al. Interlamellar CA1 network in the hippocampus. Proc. Natl. Acad. Sci. USA 111, 12919鈥?2924. https://doi.org/10.1073/pnas.1405468111 (2014).ADS聽 CAS聽 Article聽 PubMed聽Google Scholar聽 33.Sun, D. G. et al. Long term potentiation, but not depression, in interlamellar hippocampus CA1. Sci. Rep. 8, 5187. https://doi.org/10.1038/s41598-018-23369-4 (2018).ADS聽 CAS聽 Article聽 PubMed聽 PubMed Central聽Google Scholar聽 34.LeGates, T. A. et al. Aberrant light directly impairs mood and learning through melanopsin-expressing neurons. Nature 491, 594鈥?98. https://doi.org/10.1038/nature11673 (2012).ADS聽 CAS聽 Article聽 PubMed聽 PubMed Central聽Google Scholar聽 35.Yang, S., Santos, M. D., Tang, C. M., Kim, J. G. Yang, S. A postsynaptic role for short-term neuronal facilitation in dendritic spines. Front Cell Neurosci. 10, 224. https://doi.org/10.3389/fncel.2016.00224 (2016).CAS聽 Article聽 PubMed聽 PubMed Central聽Google Scholar聽 36.Yang, S. et al. Integrity of mGluR-LTD in the associative/commissural inputs to CA3 correlates with successful aging in rats. J. Neurosci. 33, 12670鈥?2678. https://doi.org/10.1523/JNEUROSCI.1086-13.2013 (2013).CAS聽 Article聽 PubMed聽 PubMed Central聽Google Scholar聽 37.Tetteh, H., Lee, J., Lee, J., Kim, J. G. Yang, S. Investigating long-term synaptic plasticity in interlamellar hippocampus CA1 by electrophysiological field recording. J. Vis. Exp. https://doi.org/10.3791/59879 (2019).Article聽 PubMed聽Google Scholar聽 38.Yang, S., Chung, J., Jin, S. H., Bao, S. Yang, S. A circuit mechanism of time-to-space conversion for perception. Hear. Res. 366, 32鈥?7. https://doi.org/10.1016/j.heares.2018.05.008 (2018).Article聽 PubMed聽Google Scholar聽 39.Wang, T. et al. iTRAQ-based proteomics analysis of hippocampus in spatial memory deficiency rats induced by simulated microgravity. J. Proteom. 160, 64鈥?3. https://doi.org/10.1016/j.jprot.2017.03.013 (2017).CAS聽 Article聽Google Scholar聽 40.Temple, M. D., Kosik, K. S. Steward, O. Spatial learning and memory is preserved in rats after early development in a microgravity environment. Neurobiol. Learn. Mem. 78, 199鈥?16 (2002).PubMed聽 Article聽Google Scholar聽 41.Sarkar, P. et al. Proteomic analysis of mice hippocampus in simulated microgravity environment. J. Proteome Res. 5, 548鈥?53 (2006).CAS聽 PubMed聽 PubMed Central聽 Article聽Google Scholar聽 42.Frigeri, A. et al. Effect of microgravity on gene expression in mouse brain. Exp. Brain Res. 191, 289鈥?00. https://doi.org/10.1007/s00221-008-1523-5 (2008).CAS聽 Article聽 PubMed聽 PubMed Central聽Google Scholar聽 43.Ranjan, A., Behari, J. Mallick, B. N. Cytomorphometric changes in hippocampal CA1 neurons exposed to simulated microgravity using rats as model. Front. Neurol. 5, 77. https://doi.org/10.3389/fneur.2014.00077 (2014).Article聽 PubMed聽 PubMed Central聽Google Scholar聽 44.Ishii, M., Tomizawa, K., Matsushita, M. Matsui, H. Exposure of mouse to high gravitation forces induces long-term potentiation in the hippocampus. Acta Med. Okayama 58, 143鈥?49 (2004).PubMed聽Google Scholar聽 45.Bojados, M. Jamon, M. The long-term consequences of the exposure to increasing gravity levels on the muscular, vestibular and cognitive functions in adult mice. Behav. Brain Res. 264, 64鈥?3. https://doi.org/10.1016/j.bbr.2014.01.018 (2014).Article聽 PubMed聽Google Scholar聽 46.Del Signore, A. et al. Hippocampal gene expression is modulated by hypergravity. Eur. J. Neurosci. 19, 667鈥?77 (2004).PubMed聽 Article聽Google Scholar聽 47.Mandillo, S. et al. Effects of acute and repeated daily exposure to hypergravity on spatial learning in mice. Neurosci. Lett. 336, 147鈥?50 (2003).CAS聽 PubMed聽 Article聽Google Scholar聽 48.Ishikawa, C. et al. Effects of gravity changes on gene expression of BDNF and serotonin receptors in the mouse brain. PLoS聽One 12, e0177833 (2017).PubMed聽 PubMed Central聽 Article聽 CAS聽Google Scholar聽 49.Horii, A. et al. Hippocampal gene expression, serum cortisol level, and spatial memory in rats exposed to hypergravity. J. Vestib. Res. 27, 209鈥?15 (2017).ADS聽 PubMed聽 Article聽Google Scholar聽 50.Mitani, K., Horii, A. Kubo, T. Impaired spatial learning after hypergravity exposure in rats. Cognit. Brain Res. 22, 94鈥?00 (2004).Article聽Google Scholar聽 51.Guinan, M. J., Horowitz, J. M. Fuller, C. A. Effects of hyperdynamic fields on input-output relationships and long-term potentiation in the rat hippocampus. J. Gravit. Physiol. 5, 31鈥?0 (1998).CAS聽 PubMed聽Google Scholar聽 52.Miller, J. D., McMillen, B. A., McConnaughey, M. M., Williams, H. L. Fuller, C. A. Effects of microgravity on brain neurotransmitter receptors. Eur. J. Pharmacol. 161, 165鈥?71 (1989).CAS聽 PubMed聽 Article聽Google Scholar聽 53.Uno, A. et al. Effects of amygdala or hippocampus lesion on hypergravity-induced motion sickness in rats. Acta Otolaryngol. 120, 860鈥?65 (2000).CAS聽 PubMed聽 Article聽Google Scholar聽 54.Horrigan, D. J., Fuller, C. A. Horowitz, J. M. Effects of hypergravic fields on serotonergic neuromodulation in the rat hippocampus. J. Gravit. Physiol. 4, 21鈥?0 (1997).CAS聽 PubMed聽Google Scholar聽 55.Bouet, V., Wubbels, R., De Jong, H. Gramsbergen, A. Behavioural consequences of hypergravity in developing rats. Dev. Brain Res. 153, 69鈥?8 (2004).CAS聽 Article聽Google Scholar聽 56.Jamon, M. The development of vestibular system and related functions in mammals: Impact of gravity. Front Integr. Neurosci. 8, 11. https://doi.org/10.3389/fnint.2014.00011 (2014).Article聽 PubMed聽 PubMed Central聽Google Scholar聽 57.Bojados, M. Jamon, M. Exposure to hypergravity during specific developmental periods differentially affects metabolism and vestibular reactions in adult C57BL/6j mice. Eur. J. Neurosci. 34, 2024鈥?034. https://doi.org/10.1111/j.1460-9568.2011.07919.x (2011).Article聽 PubMed聽Google Scholar聽 58.Wubbels, R. J. de Jong, H. A. Vestibular-induced behaviour of rats born and raised in hypergravity. Brain Res. Bull. 52, 349鈥?56 (2000).CAS聽 PubMed聽 Article聽Google Scholar聽 59.Hitier, M., Besnard, S. Smith, P. F. Vestibular pathways involved in cognition. Front Integr. Neurosci. 8, 59. https://doi.org/10.3389/fnint.2014.00059 (2014).Article聽 PubMed聽 PubMed Central聽Google Scholar聽 60.Smith, P., Geddes, L., Baek, J.-H., Darlington, C. Zheng, Y. Modulation of memory by vestibular lesions and galvanic vestibular stimulation. Front. Neurol. 1, 141 (2010).PubMed聽 PubMed Central聽 Article聽Google Scholar聽 61.Smith, P. F. et al. The effects of vestibular lesions on hippocampal function in rats. Prog. Neurobiol. 75, 391鈥?05. https://doi.org/10.1016/j.pneurobio.2005.04.004 (2005).CAS聽 Article聽 PubMed聽Google Scholar聽 62.Smith, P. F. Zheng, Y. From ear to uncertainty: Vestibular contributions to cognitive function. Front. Integr. Neurosci. 7, 84. https://doi.org/10.3389/fnint.2013.00084 (2013).Article聽 PubMed聽 PubMed Central聽Google Scholar聽 63.Stackman, R. W., Clark, A. S. Taube, J. S. Hippocampal spatial representations require vestibular input. Hippocampus 12, 291鈥?03 (2002).PubMed聽 PubMed Central聽 Article聽Google Scholar聽 64.Rockland, K. S. Van Hoesen, G. W. Some temporal and parietal cortical connections converge in CA1 of the primate hippocampus. Cereb. Cortex 9, 232鈥?37 (1999).CAS聽 PubMed聽 Article聽Google Scholar聽 65.Horii, A., Russell, N. A., Smith, P. F., Darlington, C. L. Bilkey, D. K. Vestibular influences on CA1 neurons in the rat hippocampus: An electrophysiological study in vivo. Exp. Brain Res. 155, 245鈥?50 (2004).PubMed聽 Article聽Google Scholar聽 66.Cuthbert, P. C., Gilchrist, D. P., Hicks, S. L., MacDougall, H. G. Curthoys, I. S. Electrophysiological evidence for vestibular activation of the guinea pig hippocampus. NeuroReport 11, 1443鈥?447 (2000).CAS聽 PubMed聽 Article聽Google Scholar聽 67.Zucker, R. S. Regehr, W. G. Short-term synaptic plasticity. Annu. Rev. Physiol. 64, 355鈥?05. https://doi.org/10.1146/annurev.physiol.64.092501.114547 (2002).CAS聽 Article聽 PubMed聽Google Scholar聽 68.Katz, B. Miledi, R. Tetrodotoxin-resistant electric activity in presynaptic terminals. J. Physiol. 203, 459鈥?87 (1969).CAS聽 PubMed聽 PubMed Central聽 Article聽Google Scholar聽 69.Chesselet, M.-F. Presynaptic regulation of neurotransmitter release in the brain: Facts and hypothesis. Neuroscience 12, 347鈥?75 (1984).CAS聽 PubMed聽 Article聽Google Scholar聽 70.Yang, S. et al. Beta-arrestin-dependent dopaminergic regulation of calcium channel activity in the axon initial segment. Cell Rep. 16, 1518鈥?526. https://doi.org/10.1016/j.celrep.2016.06.098 (2016).CAS聽 Article聽 PubMed聽 PubMed Central聽Google Scholar聽 71.Borisova, T., Krisanova, N. Himmelreich, N. Exposure of animals to artificial gravity conditions leads to the alteration of the glutamate release from rat cerebral hemispheres nerve terminals. Adv. Sp. Res. 33, 1362鈥?367. https://doi.org/10.1016/j.asr.2003.09.039 (2004).ADS聽 CAS聽 Article聽Google Scholar聽 72.Noh, W., Lee, M., Kim, H. J., Kim, K.-S. Yang, S. Hypergravity induced disruption of cerebellar motor coordination. Sci. Rep. 10, 4452. https://doi.org/10.1038/s41598-020-61453-w (2020).ADS聽 CAS聽 Article聽 PubMed聽 PubMed Central聽Google Scholar聽 73.Beraneck, M., Bojados, M., LeS茅ach, A., Jamon, M. Vidal, P.-P. Ontogeny of mouse vestibulo-ocular reflex following genetic or environmental alteration of gravity sensing. PLoS聽One 7, 40414 (2012).ADS聽 Article聽 CAS聽Google Scholar聽 74.Mirzoev, T. et al. Divergent anabolic signalling responses of murine soleus and tibialis anterior muscles to chronic 2g hypergravity. Sci. Rep. 7, 1鈥? (2017).CAS聽 Article聽Google Scholar聽 75.Gnyubkin, V. et al. Effects of chronic hypergravity: From adaptive to deleterious responses in growing mouse skeleton. J. Appl. Physiol. 119, 908鈥?17 (2015).CAS聽 PubMed聽 Article聽Google Scholar聽 76.Baker, K. B. Kim, J. J. Effects of stress and hippocampal NMDA receptor antagonism on recognition memory in rats. Learn. Memory 9, 58鈥?5 (2002).Article聽Google Scholar聽 77.Cohen, S. J. et al. The rodent hippocampus is essential for nonspatial object memory. Curr. Biol. 23, 1685鈥?690 (2013).CAS聽 PubMed聽 PubMed Central聽 Article聽Google Scholar聽 78.de Lima, M. N., Luft, T., Roesler, R. Schr枚der, N. Temporary inactivation reveals an essential role of the dorsal hippocampus in consolidation of object recognition memory. Neurosci. Lett. 405, 142鈥?46 (2006).PubMed聽 Article聽 CAS聽Google Scholar聽 79.Hammond, R. S., Tull, L. E. Stackman, R. W. On the delay-dependent involvement of the hippocampus in object recognition memory. Neurobiol. Learn. Mem. 82, 26鈥?4 (2004).PubMed聽 Article聽Google Scholar聽 80.de Lima, M. N. M., Laranja, D. C., Bromberg, E., Roesler, R. Schr枚der, N. Pre-or post-training administration of the NMDA receptor blocker MK-801 impairs object recognition memory in rats. Behav. Brain Res. 156, 139鈥?43 (2005).PubMed聽 Article聽 CAS聽Google Scholar聽 81.Hunt, D. L. Castillo, P. E. Synaptic plasticity of NMDA receptors: Mechanisms and functional implications. Curr. Opin. Neurobiol. 22, 496鈥?08. https://doi.org/10.1016/j.conb.2012.01.007 (2012).CAS聽 Article聽 PubMed聽 PubMed Central聽Google Scholar聽 82.Sidorov, M. S., Kaplan, E. S., Osterweil, E. K., Lindemann, L. Bear, M. F. Metabotropic glutamate receptor signaling is required for NMDA receptor-dependent ocular dominance plasticity and LTD in visual cortex. Proc. Natl. Acad. Sci. USA 112, 12852鈥?2857. https://doi.org/10.1073/pnas.1512878112 (2015).ADS聽 CAS聽 Article聽 PubMed聽Google Scholar聽 83.Yang, S., Yang, S., Park, J. S., Kirkwood, A. Bao, S. Failed stabilization for long-term potentiation in the auditory cortex of FMR1 knockout mice. PLoS聽One 9, e104691. https://doi.org/10.1371/journal.pone.0104691 (2014).ADS聽 CAS聽 Article聽 PubMed聽 PubMed Central聽Google Scholar聽 84.Noh, W., Pak, S., Choi, G., Yang, S. Yang, S. Transient potassium channels: Therapeutic targets for brain disorders. Front Cell. Neurosci. 13, 265. https://doi.org/10.3389/fncel.2019.00265 (2019).CAS聽 Article聽 PubMed聽 PubMed Central聽Google Scholar聽 85.Tetteh, H., Lee, M., Lau, C. G., Yang, S. Yang, S. Tinnitus: Prospects for pharmacological interventions with a seesaw model. Neuroscientist 24, 353鈥?67. https://doi.org/10.1177/1073858417733415 (2018).CAS聽 Article聽 PubMed聽Google Scholar聽 Download referencesAcknowledgementsWe thank Sungchil Yang for constructive comments on this research. This research was supported by Incheon National University (International Cooperative) Research Grant and the Ministry of Education (no. 2016R1D1A1B04930938) for Sunggu Yang, National Research Foundation of Korea (NRF) funded by the Ministry of Education (No. 2018R1A6A1A03025523) for Kyu-Sung Kim.Author informationAuthor notesThese authors contributed equally: Jinho Lee and Doohyeong Jang.AffiliationsDepartment of Nano-Bioengineering, Incheon National University, Incheon, South KoreaJinho Lee,聽Doohyeong Jang,聽Hyerin Jeong聽 聽Sunggu YangDepartment of Otorhinolaryngology-Head and Neck Surgery, Inha University, College of Medicine, Incheon, South KoreaKyu-sung KimInha Institute of Aerospace Medicine, Incheon, South KoreaKyu-sung KimAuthorsJinho LeeView author publicationsYou can also search for this author in PubMed聽Google ScholarDoohyeong JangView author publicationsYou can also search for this author in PubMed聽Google ScholarHyerin JeongView author publicationsYou can also search for this author in PubMed聽Google ScholarKyu-sung KimView author publicationsYou can also search for this author in PubMed聽Google ScholarSunggu YangView author publicationsYou can also search for this author in PubMed聽Google ScholarContributionsS.Y. and K.-s.K. designed and conceptualized the work. J.L., D.J., and H.J. performed electrophysiological and behavioral experiments and analyzed data. S.Y., K.-s.K., and J.L. wrote and revised the manuscript. All authors reviewed the manuscript.Corresponding authorsCorrespondence to Kyu-sung Kim or Sunggu Yang.Ethics declarations Competing interests The authors declare no competing interests. Additional informationPublisher\'s noteSpringer Nature remains neutral with regard to jurisdictional claims in published maps and institutional affiliations.Rights and permissions Open Access This article is licensed under a Creative Commons Attribution 4.0 International License, which permits use, sharing, adaptation, distribution and reproduction in any medium or format, as long as you give appropriate credit to the original author(s) and the source, provide a link to the Creative Commons licence, and indicate if changes were made. The images or other third party material in this article are included in the article\'s Creative Commons licence, unless indicated otherwise in a credit line to the material. If material is not included in the article\'s Creative Commons licence and your intended use is not permitted by statutory regulation or exceeds the permitted use, you will need to obtain permission directly from the copyright holder. To view a copy of this licence, visit http://creativecommons.org/licenses/by/4.0/. Reprints and PermissionsAbout this articleCite this articleLee, J., Jang, D., Jeong, H. et al. Impairment of synaptic plasticity and novel object recognition in the hypergravity-exposed rats. Sci Rep 10, 15813 (2020). https://doi.org/10.1038/s41598-020-72639-7Download citationReceived: 12 December 2019Accepted: 24 August 2020Published: 25 September 2020DOI: https://doi.org/10.1038/s41598-020-72639-7 CommentsBy submitting a comment you agree to abide by our Terms and Community Guidelines. If you find something abusive or that does not comply with our terms or guidelines please flag it as inappropriate. Sign up for the Nature Briefing newsletter 鈥?what matters in science, free to your inbox daily.
本文链接: https://www.ebiomall.com/b105-molecular/info-12395.html
免责声明 本文仅代表作者个人观点,与本网无关。其创作性以及文中陈述文字和内容未经本站证实,对本文以及其中全部或者部分内容、文字的真实性、完整性、及时性本站不做任何保证或承诺,请读者仅作参考,并请自行核实相关内容。
版权声明 未经蚂蚁淘授权不得转载、摘编或利用其他方式使用上述作品。已经经本网授权使用作品的,应该授权范围内使用,并注明“来源:蚂蚁淘”。违反上述声明者,本网将追究其相关法律责任。
相关文章
1970-01-01
2008-04-20
2011-10-26
2013-01-09
2014-09-06
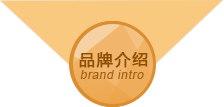
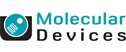
▍
品牌分类
▍
官网分类
▍
品牌问答
暂无品牌问答