新闻动态
AWP1/ZFAND6 Functions in Pex5 Export by Interacting with Cys...
2024-04-27
Free Access AWP1/ZFAND6 Functions in Pex5 Export by Interacting with Cys-Monoubiquitinated Pex5 and Pex6 AAA ATPase Non Miyata, Department of Biology, Faculty of Sciences, Kyushu University, Fukuoka, 812-8581, JapanSearch for more papers by this authorKanji Okumoto, Department of Biology, Faculty of Sciences, Kyushu University, Fukuoka, 812-8581, Japan Graduate School of Systems Life Sciences, Kyushu University Graduate School, Fukuoka, 812-8581, JapanSearch for more papers by this authorSatoru Mukai, Department of Biology, Faculty of Sciences, Kyushu University, Fukuoka, 812-8581, JapanSearch for more papers by this authorMasafumi Noguchi, Graduate School of Systems Life Sciences, Kyushu University Graduate School, Fukuoka, 812-8581, JapanSearch for more papers by this authorYukio Fujiki, Corresponding Author Department of Biology, Faculty of Sciences, Kyushu University, Fukuoka, 812-8581, Japan Graduate School of Systems Life Sciences, Kyushu University Graduate School, Fukuoka, 812-8581, Japan JST, CREST, Chiyoda Tokyo, 102-0075, JapanYukio Fujiki, yfujiki@kyudai.jpSearch for more papers by this author Give accessShare full text accessShare full-text accessPlease review our Terms and Conditions of Use and check box below to share full-text version of article.I have read and accept the Wiley Online Library Terms and Conditions of UseShareable LinkUse the link below to share a full-text version of this article with your friends and colleagues. Learn more.Copy URLShare a linkShare onEmailFacebookTwitterLinked InRedditWechat Abstract During biogenesis of the peroxisome, a subcellular organelle, the peroxisomal-targeting signal 1 (PTS1) receptor Pex5 functions as a shuttling receptor for PTS1-containing peroxisomal matrix proteins. However, the precise mechanism of receptor shuttling between peroxisomes and cytosol remains elusive despite the identification of numerous peroxins involved in this process. Herein, a new factor was isolated by a combination of biochemical fractionation and an in vitro Pex5 export assay, and was identified as AWP1/ZFAND6, a ubiquitin-binding NF-κB modulator. In the in vitro Pex5 export assay, recombinant AWP1 stimulated Pex5 export and an anti-AWP1 antibody interfered with Pex5 export. AWP1 interacted with Pex6 AAA ATPase, but not with Pex1–Pex6 complexes. Preferential binding of AWP1 to the cysteine-ubiquitinated form of Pex5 rather than to unmodified Pex5 was mediated by the AWP1 A20 zinc-finger domain. Inhibition of AWP1 by RNA interference had a significant effect on PTS1-protein import into peroxisomes. Furthermore, in AWP1 knock-down cells, Pex5 stability was decreased, similar to fibroblasts from patients defective in Pex1, Pex6 and Pex26, all of which are required for Pex5 export. Taken together, these results identify AWP1 as a novel cofactor of Pex6 involved in the regulation of Pex5 export during peroxisome biogenesis. To elucidate the hierarchy of events involved in the organized biogenesis of over a dozen intracellular organelles, the peroxisome, a single membrane-bounded essential organelle 1, has proven to be a useful model of biogenesis in mammalian, plant and yeast systems 2-5. In addition, peroxisomal protein import is in and of itself a fascinating process because it differs so much from that of other organelles 2-5. Zellweger syndrome and neonatal adrenoleukodystrophy in humans are fatal genetic disorders of peroxisome biogenesis. They are autosomal recessive in nature and map to 13 complementation groups 6-8. Significant and rapid progress has been made in the last two decades in our understanding of the biogenesis of peroxisomes and in delineating the functions of 13 PEX genes responsible for the peroxisome biogenesis disorders 3, 9-13. Peroxisomal matrix proteins are synthesized on free polyribosomes and post-translationally imported into peroxisomes 14. The majority of peroxisomal matrix proteins contain a type 1 peroxisome-targeting signal (PTS1) at their C-terminus consisting of a tripeptide SKL motif 15, 16. The PTS1 receptor Pex5 plays a central role in the translocation of peroxisomal matrix proteins 17-19. Pex5 recognizes newly synthesized PTS1-containing proteins in the cytosol and transports them into the peroxisomal matrix. This process consists of several distinct steps involving a number of proteins termed peroxins located on the peroxisome, including Pex14, Pex13, Pex2, Pex10 and Pex12 20-27. Pex14 and Pex13 are believed to form the initial Pex5 docking complex 17, 28, 29, which has a mass of approximately 800 kDa 30. After docking onto the peroxisome, Pex5 transits to the RING complex, which consists of the RING peroxins Pex2, Pex10 and Pex12 26-28. In the last step of the protein import reaction, Pex5 exits from the peroxisome and shuttles back to the cytosol for the next round of protein import 30-33. Pex5 export from peroxisomes is dependent on ATP hydrolysis 30, 32, 33 and the AAA ATPase family peroxins Pex1 and Pex6, as well as the Pex1–Pex6 recruiter, Pex26 (Pex15 in yeast) 30, 32. Pex5 export is also believed to require ubiquitination of a highly conserved cysteine residue in the N-terminal region of Pex5 34-36. Cysteine-linked ubiquitination (cys-ubiquitination) of Pex5 is catalyzed by an E2 enzyme, UbcH5 in mammalian cells 37 and Pex4 in yeast 35, 38. The three RING peroxins have been shown to possess E3 ligase activity 39, 40, which supports a model in Saccharomyces cerevisiae in which Pex12 mediates Pex5 ubiquitination with Pex4 functioning as the E2 enzyme. We previously demonstrated that the export of Pex5 is dependent on the presence of a cellular cytosolic fraction 30, suggesting that cytosolic factor(s) are more likely involved in the Pex5 export reaction. To elucidate the mechanism of protein import into peroxisomes, we set out to isolate and characterize these putative cytosolic factors involved in Pex5 export. Using a combination of chromatographic fractionation and an in vitro Pex5 export assay, we isolated and identified an A20/AN1 zinc-finger-domain-containing protein termed AWP1/ZFAND6 41 as a putative cytosolic Pex5 export protein. A20/AN1 zinc-finger-domain-containing proteins function in immune responses 42, 43 and stress tolerance 44. AWP1 specifically has been shown to modulate NF-κB activity 42, 43. AWP1 bound to Pex6 and cys-ubiquitinated Pex5. In AWP1 knock-down cells, PTS1-protein import was decreased, suggesting that AWP1 functions as a novel cofactor of Pex6 involved in Pex5 export from peroxisomes. Shuttling of Pex5 between the cytosol and peroxisomes is essential for import of PTS1- and PTS2-containing proteins 30, 32, 45. Pex5 is exported in an ATP-dependent and N-ethylmaleimide (NEM)-sensitive manner 30, 46, consistent with the findings that Pex5 export requires the AAA-ATPase activity of Pex1 and Pex6 and the Pex1/Pex6 recruiter Pex26 7, 30 [Pex15 in yeast 32 and APEM9 in plants 47]. On the basis of a previous finding that Pex5 export was dependent on a cytosolic cellular fraction, we sought the identity of the important cytosolic factor(s) involved in Pex5 export 30. Cytosolic factor(s) responsible for Pex5 export were identified and purified using an in vitro Pex5 export assay, as previously described, with some modifications (described in Materials and Methods). Briefly, an 35S-Pex5 import reaction was performed at 26°C for 30 min using a post-heavy mitochondrial supernatant fraction from rat liver. The organelle fraction was isolated, resuspended in homogenizing buffer and then divided into aliquots and stored at −80°C until use. An 35S-Pex5 export assay was performed with gently thawed organelle fractions (peroxisomes) from the 35S-Pex5 import reaction, as described. 35S-Pex5 in the supernatant fraction, representing exported 35S-Pex5 30, was increased in the presence of a cytosolic fraction added to the export assay compared to export assay buffer alone (Figure 1A). These results demonstrated that the cytosol-dependent Pex5 export seen with freshly isolated peroxisomes 30 was efficiently reproduced using reconstituted peroxisomes. Pex5 export is augmented by cytosolic proteins. A) An in vitro Pex5 export assay was performed using 35S-Pex5-imported peroxisomes. 35S-Pex5-imported post-heavy mitochondrial supernatant fractions were incubated at 26°C for 30 min with export assay buffer (lane 1) or rat liver cytosol (lane 2), in addition to an ATP-regenerating system. After incubation, reaction mixtures were centrifuged at 20 000 ×g for 30 min at 4°C. The resulting supernatant fractions were analyzed by SDS–PAGE using a 10% polyacrylamide gel. Exported 35S-Pex5 was detected using a Fujix FLA-5000 autoimaging analyzer. Levels of exported 35S-Pex5 are shown as fold-increase relative to buffer. B) Rat liver cytosolic proteins were precipitated with 30% (wt/vol)-saturated ammonium sulfate. The precipitate was dissolved in 0.15 m KCl and assayed for 35S-Pex5 export activity (lane 2), after which it was sequentially passed through a HiTrap Q and HiTrap SP column. The respective flow-through fractions (F-T) were also assayed for 35S-Pex5 export activity (lanes 3 and 4). Solid and open bars indicate exported 35S-Pex5 levels determined as in A and protein concentration (mg/mL), respectively. C) Left panel, rat liver cytosolic proteins precipitated with 30% (wt/vol)-saturated ammonium sulfate, as in B, were dissolved in 0.15 m KCl and loaded onto a HiTrap Q column. After thorough washing with 0.15 m KCl, bound proteins were eluted into 11 fractions with a 0.15–0.6 M KCl gradient solution. Fractions 5–11 were assessed for 35S-Pex5p export activity as in A. Right panel, fraction 8, showing the highest Pex5 export activity (left panel), was further fractionated by ultracentrifugation on a 5–30% sucrose gradient. The gradients were collected into 10 tubes from the base of the gradient. Each fraction was verified in the 35S-Pex5 export assay as in A. Size markers were as follows: bovine catalase (230 kDa), bovine LDH (140 kDa), bovine serum albumin (66 kDa) and bovine carbonic anhydrase (29 kDa), indicated by downward arrowheads. D) The High-Trap Q flow-through fraction was fractionated by ultracentrifugation on a sucrose gradient as in C and each fraction was verified for 35S-Pex5 export activity. Cytosolic proteins were fractionated by chromatography and then the individual fractions were assayed for their ability to augment Pex5 export in the in vitro35S-Pex5 export assay. Proteins from rat liver cytosol were first precipitated with a 30% saturated (NH4)2SO4 solution, dissolved in 20 mm Tris–HCl, pH 7.0 containing 1 mm DTT, and then adjusted to 0.15 m KCl by gel-filtration through an Econo Pac 10 DG column. 35S-Pex5 export activity in the 30%-saturated (NH4)2SO4-precipitated preparation was elevated several fold compared to buffer alone (Figure 1B, lanes 1 and 2), suggesting that the (NH4)2SO4-precipitated cytosolic fraction contained a potential 35S-Pex5 export factor. The (NH4)2SO4-precipitated cytosolic fraction was sequentially applied onto an anion-exchange column (HiTrap Q) and then a cation-exchange column (HiTrap SP), and the flow-through fractions from each column were assessed by 35S-Pex5 export assay. In the flow-through fraction of the HiTrap Q (HiTrap Q F-T) column, approximately 60% 35S-Pex5 export activity was present (Figure 1B, lane 3) relative to the (NH4)2SO4-precipitated fraction (lane 2), which indicated that some of the proteins with Pex5 export-enhancing activity were retained by the HiTrap Q column. Most of the Pex5 export-enhancing activity in the HiTrap Q flow-through fraction was retained in the HiTrap SP column, with partial activity detected in the flow-through fraction (lane 4). These results suggested the presence of at least two distinct factors with Pex5 export-enhancing activity in the (NH4)2SO4-precipitated cytosolic fraction that were separable by their differential retention properties in the HiTrap Q and HiTrap SP columns. The HiTrap Q-bound (Figure 1C, left panel, fraction 8) and flow-through (Figure 1B) fractions were further fractionated by ultracentrifugation on a sucrose density gradient. After centrifugation, the gradient was fractionated into 10 fractions and each fraction was assayed for 35S-Pex5 export activity. From the HiTrap Q-bound fraction, 35S-Pex5 export activity was enriched in a high sucrose-density region, with a peak in fraction 4 (Figure 1C, right panel). In contrast, in the HiTrap Q flow-through fraction, 35S-Pex5 export activity was detected in a low sucrose-density region with a peak in fractions 8 and 9 (Figure 1D). These results suggested that there are several Pex5 export-enhancing factors in rat liver cytosol. The scheme for purifying the Pex5 export-enhancing factor from the HiTrap Q flow-through fraction is indicated in Figure 2A. Proteins in the HiTrap Q flow-through fraction were loaded onto the HiTrap SP column. The bound fraction was eluted with a 0.15–0.6 m KCl gradient and collected into 10 tubes. Pex5 export activity was detected in fractions 1 and 2 (Figure 2B). Fraction 1 was subjected to sucrose density gradient centrifugation, from which fractions 6–10 contained relatively higher 35S-Pex5 export specific activity (Figure 2C, upper panel). Each of these fractions was concentrated by trichloroacetic acid precipitation and then subjected to SDS–PAGE and silver staining (Figure 2C, lower panel). Two major proteins with molecular masses of approximately 40 and 30 kDa were detectable in fractions 8 and 9, both of which exhibited higher 35S-Pex5 export activity (Figure 2C). Following in-gel digestion and mass spectrometry, the two proteins were identified as AWP1/ZFAND6 (223 amino acids; 24.0 kDa), a zinc-finger-domain-containing NF-κB modulator 41-43, and CDV3a/TPP32, a substrate of the Abl tyrosine kinase 48 (Figure 2D). AWP1 purified from rat liver cytosol (Figure 2B,C) and recombinant mouse AWP1 (Figure 3B) both migrated as lower molecular mass proteins on SDS–PAGE gels relative to their estimated molecular mass, as has been reported for human AWP1 41. Purification and identification of a Pex5 export factor. A) Flow diagram for the purification of a Pex5 export factor from rat liver cytosol. Abbreviation: TCA ppt., precipitation with trichloroacetic acid. B) Rat liver cytosol was fractionated according to the protocol shown in A (see Materials and Methods). The flow-through fraction from the HiTrap Q column (Figure 1B) was loaded onto a HiTrap SP column. Bound proteins were eluted into 10 fractions with a 0.15–0.6 m KCl gradient solution and assessed for Pex5p export activity, as in Figure 1A. Solid and open bars indicate 35S-Pex5p-export activity relative to that of the cytosol and protein concentration, respectively. C) Fraction 1, which showed the highest activity in the 35S-Pex5 export assay (B), was further fractionated by ultracentrifugation on a 5–30% sucrose gradient. The gradient was collected in 10 tubes and fractions 6–10 were concentrated by trichloroacetic-acid precipitation, after which one third of each aliquot was analyzed by SDS–PAGE using a 12% polyacrylamide gel. Proteins were visualized by silver staining. Open arrowhead indicates a protein band that co-fractionated with Pex5 export activity. Molecular mass markers (Precision Plus Protein Standards, BioRad) are on the left. D) Deduced amino acid sequence of rat AWP1/ZFAND6. Peptides indicated by bold one-letter amino acids were identified by mass spectrometry. AWP1 is involved in Pex5 export. A) Schematic representation of mouse AWP1. Black boxes indicate the A20-type and AN1-type zinc-finger domains; closed and open arrowheads indicate the positions of the active-center cysteine residues in the A20- and AN1-type zinc-finger domains (C30 and C180, respectively); numbers designate amino acid positions of AWP1. B) Bacterially expressed recombinant AWP1 (rAWP1) variants were purified and analyzed by SDS–PAGE. Lanes: 1, wild-type (Wt) rAWP1; 2, AWP1-C30S. Proteins were visualized by Coomassie Brilliant Blue (CBB) staining. Open arrowhead indicates wild-type rAWP1 and its mutant. Molecular mass markers (Low-Range Prestained SDS–PAGE Standards, BioRad) are on the left. C) An 35S-Pex5 export assay was performed using a cytosolic fraction (150 µg, lane 2), buffer (lane 3), wild-type rAWP1 (lanes 4–6) and rAWP1-C30S (lanes 7–9), as indicated. Exported 35S-Pex5 was analyzed as in Figure 1A. A portion (one quarter) of the 35S-Pex5-imported peroxisomal fraction used for the export assay was loaded in lane 1. Data represent means ± SD of three independent experiments (lower panel). *p 0.05. D) An 35S-Myc3-Pex5 export assay was performed using buffer (lane 2), cytosolic fraction (lane 3), cytosolic fraction treated with an anti-AWP1 antibody (lane 4), and cytosolic fraction treated with preimmune serum (lane 5). A portion (one quarter) of the 35S-Myc3-Pex5-imported peroxisomal fraction used in the export assay was loaded in lane 1. Exported 35S-Myc3-Pex5 was quantified and represented as in (C). Data represent the means ± SD of three independent experiments (lower panel). AWP1 contains two zinc-finger domains, an A20 type and an AN1 type 41-43 (Figure 3A). The A20-type zinc-finger domain, which is a class of Cys2/Cys2-type zinc finger, plays an important role in NF-κB regulation 42, 43 and the recognition of poly-ubiquitinated proteins 43, 49. To determine whether AWP1 stimulated 35S-Pex5 export in vitro, recombinant mouse AWP1 (identical in primary sequence to rat AWP1) and an AWP1 mutant harboring a C30S point mutation of the conserved cysteine of the A20-type zinc-finger domain (rAWP1 and rAWP1-C30S, respectively) were purified from Escherichia coli (Figure 3B). In the 35S-Pex5 export assay, the addition of 0.12 or 0.24 µg/mL of rAWP1 stimulated 35S-Pex5 export at a low level compared to the control reaction with buffer only (Figure 3C, lanes 3–5). The addition of 0.6 µg/mL of rAWP1 resulted in a twofold increase in 35S-Pex5 export activity relative to the control (lane6). In contrast, 35S-Pex5 export was not increased by rAWP1-C30S at any concentration (lanes 7–9). To confirm that AWP1 was involved in Pex5 export, the export assay was repeated using cytosolic fractions treated with an anti-AWP1 antiserum, or preimmune serum as a control. Stimulation of 35S-Pex5 export was significantly lower when a cytosolic fraction treated with anti-AWP1 antibodies was employed instead of the untreated fraction (Figure 3D, lanes 3 and 4). 35S-Pex5 export was not affected by treatment of the cytosol with preimmune serum (lane 5). Similar results were obtained with rat liver cytosolic fractions that were preincubated at 4°C for 1 h with anti-AWP1 antiserum (data not shown). Together, these results strongly suggested that AWP1 is at least one of the cytosolic factors associated with Pex5 export. We and other groups have reported that the AAA ATPase family peroxins Pex1 and Pex6 are required for Pex5 export 30, 32. To determine whether AWP1 interacted with either Pex1 or Pex6 to modulate their function in Pex5 export, wild-type CHO-K1 cells were transfected with expression vectors for FLAG-tagged AWP1 (FL-AWP1) and hemagglutinin-tagged Pex1 (PEX1-HA), PEX6-HA, or both. Cell lysates were subjected to immunoprecipitation using an anti-FLAG antibody followed by immunoblot analysis. Pex6-HA and a small amount of Pex1-HA co-immunoprecipitated with AWP1 (Figure 4A, lanes 7 and 8), which suggested that AWP1 interacts with Pex6 and weakly with Pex1. Mammalian Pex26, and in yeast, Pex15, recruit Pex1/Pex6 complexes to peroxisomes through binding to Pex6 7, 50. However, under the same experimental conditions, where nearly equal amounts of Pex1-HA and Pex6-HA co-immunoprecipitated with FL-Pex26 (Figure 4B, lane 5) 7, Pex6 and a barely detectable level of Pex1 co-immunoprecipitated with FL-AWP1 (lane 6). AWP1 interacts with the Pex6 AAA ATPase. A) Proteins, as indicated, were transiently expressed in CHO-K1 cells (approximately 5 × 105 each). Whole-cell lysates were subjected to immunoprecipitation with anti-Flag antibody followed by immunoblot using an anti-HA antibody or an anti-Flag antibody. A portion (one fortieth) of the whole-cell lysate used in the assay was loaded in lanes 1–4. B) Co-immunoprecipitation assays including Flag-Pex26 (FL-Pex26) were performed as in A. Pex1-HA and Pex6-HA were detected with an anti-HA antibody; FL-Pex26 and Flag-AWP1 (FL-AWP1) were detected using an anti-Flag antibody. A portion of whole-cell lysate was loaded as in A. C) Interaction between Pex6-HA and FL-AWP1 variants, including FL-AWP1-C30S and FL-AWP1-C180S, was assessed by co-immunoprecipitation assay as in A. D) Interaction between FL-AWP1 and Pex6-HA mutants harboring point mutations in the Walker motifs was verified by immunoprecipitation as in A. E) Interaction between FL-AWP1 and a Pex6-HA mutant carrying mutations in both A1 and A2 was investigated as in A. F) CHO-K1 cells transiently expressing either Pex6-HA or Pex6-HA and FL-AWP1 were homogenized and fractionated into cytosol (S) and organelle (P) fractions. Organelle proteins were solubilized with buffer containing 0.5% NP-40 and the cytosol fraction was adjusted to the same buffer conditions. Immunoprecipitation with an anti-Flag antibody was performed as in A. Endogenous Pex14 and LDH were detected by their respective specific antibodies. To determine whether the A20- and AN1-zinc-finger domains of AWP1 were required for the interaction with Pex6, FL-AWP1 variants harboring serine substitutions of the active-site cysteines in the zinc-finger domains (FL-AWP1-C30S and FL-AWP1-C180S, respectively) (see Figure 3A) were co-expressed with Pex6-HA in CHO-K1 cells. Pex6-HA was detected at significantly lower levels in association with FL-AWP1-C180S compared to wild-type FL-AWP1 (Figure 4C, lanes 6 and 8), while the association of Pex6-HA with FL-AWP1-C30S was undetectable (lane 7). These results suggested that both zinc-finger domains of AWP1 play a pivotal role in the interaction with Pex6. Furthermore, the A20-type domain may be more important for the interaction with Pex6 than the AN1-type domain, given that FL-AWP1-C180S expression was nearly twofold lower compared to FL-AWP1-Wt and FL-AWP1-C30S. Pex6 contains three Walker motifs: A1, A2 and B2 51. To investigate whether the Walker motifs of Pex6 were involved in its interaction with AWP1, three Walker-motif mutants of Pex6 were co-expressed in CHO-K1 cells along with FL-AWP1, following which cell extracts were prepared and subjected to co-immunoprecipitation and immunoblot analysis (Figure 4D). The Pex6 Walker-motif variants co-immunoprecipitated with FL-AWP1 at approximately 30–50% the level of wild-type Pex6 (Figure 4D, lanes 6–10). Furthermore, FL-AWP1 binding to Pex6 was more severely affected by mutation of both A1 and A2 (Figure 4E). These results suggested that the ATPase activity of Pex6 modulates its interaction with AWP1. AWP1-stimulated Pex5 export in the in vitro assay was initiated with only rAWP1 and organelle-associated proteins (Figure 3). Given that the Pex5 export-enhancing activity of AWP1 was dependent on its ability to interact with Pex6, we speculated that AWP1 interacts with Pex6 on the peroxisome rather than in the cytosol. To verify this, we performed immunoprecipitation assays using cytosolic (S) and organelle (P) fractions from CHO-K1 cells co-expressing FL-AWP1 and PEX6-HA. The organelle and cytosolic markers Pex14 and lactate dehydrogenase (LDH) were detected exclusively in the P and S fractions, respectively (Figure 4F, lanes 1–4), confirming the adequate separation of cytosol and organelles. FL-AWP1 was detected mostly in the S fraction, with a low level also apparent in the P fraction (lanes 2 and 4). Pex6-HA co-immunoprecipitated with FL-AWP1 from both the S and P fractions (lanes 6 and 8), suggesting that FL-AWP1 interacts with Pex6-HA on organelles as well as in the cytosol, despite lower levels of FL-AWP1 in the organelle fraction. Endogenous Pex6 localizes predominantly to peroxisomes 52. Thus, it seems likely that AWP1 regulates the export of Pex5 via an interaction with Pex6 on the peroxisome. CDV3a/TPP32, which co-purified with AWP1 as a putative Pex5 export-enhancing factor from rat liver cytosol (see Figure 2B), failed to bind to Pex1, Pex6 and AWP1 (data not shown). Furthermore, in CDV3a/TPP32 knock-down cells, peroxisomal matrix protein import was similar to that in wild-type cells (data not shown). Hence, CDV3a/TPP32 is not likely to be a critical factor involved in Pex5 export. According to the current model of Pex5 shuttling, Pex5 is mono- and poly-ubiquitinated on the peroxisome for export and quality control, respectively 37, 38, 53. However, it remains unclear how and when ubiquitinated Pex5 is recognized and transported to its destination. The A20 zinc-finger domain of AWP1 reportedly interacts with poly-ubiquitin chains 43. To investigate whether AWP1 interacted with ubiquitinated proteins in vivo, Flag-AWP1-Wt (FL-AWP1-Wt) and Flag-AWP1-C30S (FL-AWP1-C30S) were separately expressed in CHO-K1 cells. Poly-ubiquitinated proteins were specifically co-immunoprecipitated with FL-AWP1-Wt, but not FL-AWP1-C30S (Figure 5A, lanes 4–6). In addition, an in vitro binding assay was performed using rAWP1 and mono-ubiquitin (Ub)-conjugated beads, or beads conjugated to mono-SUMO, a Ub-like protein. Wild-type rAWP1-Wt bound to Ub-conjugated beads, but not to SUMO-conjugated beads (Figure 5B, lanes 3 and 5), while rAWP1-C30S did not bind to either (lanes 4 and 6). Similarly, synthetic K48-linked poly-Ub chains and poly-ubiquitinated proteins from HeLa cell lysate bound to AWP1-Flag-Wt, but not to AWP1-Flag-C30S or control anti-Flag immunoglobulin G (IgG)-beads (Figure 5C). There was also a significant level of binding of mono-Ub to AWP1-Flag-Wt (Figure 5C, upper panel, lane 3; lower panel, lane 7), which was consistent with the results in Figure 5B. These results strongly suggested that AWP1 binds directly to both poly- and mono-Ub via the A20-type zinc-finger domain. AWP1 interacts with poly-ubiquitin chain and mono-ubiquitin. A) Flag-AWP1-Wt (FL-AWP1-Wt) (lanes 2 and 5) and Flag-AWP1-C30S (FL-AWP1-C30S) (lanes 3 and 6) were separately expressed in CHO-K1 cells. Whole-cell lysates, including control CHO-K1 (lanes 1 and 4), were subjected to immunoprecipitation with anti-Flag antibody followed by immunoblot using anti-ubiquitin (upper panel) and -Flag (lower panel) antibodies. Input (lanes 1–3) consisted of a portion (one fortieth) of the whole-cell lysate used for immunoprecipitation. Lanes: 1 and 4, CHO-K1; 2 and 5, CHO-K1 expressing FL-AWP1-Wt; 3 and 6, CHO-K1 expressing FL-AWP1-C30S. B) Recombinant AWP1-Wt (lanes 1, 3 and 5) and AWP1-C30S (lanes 2, 4 and 6) (0.5 µg each) were incubated with mono-ubiquitin-conjugated beads (lanes 3 and 4) and mono-SUMO-conjugated beads (lanes 5 and 6). After thorough washing, rAWP1 bound to beads was detected with an anti-AWP1 antibody. Input, 25 ng of each rAWP1 variants. C) K48-linked poly-ubiquitin chains (upper panel) and HeLa cell lysates (lower panel) were incubated with anti-Flag IgG-agarose beads conjugated with recombinant AWP1-Flag-Wt (Wt, lanes 3 and 7) and AWP1-Flag-C30S (C30S, lanes 4 and 8) or with beads alone (FL beads, 2 and 6). Proteins bound to the beads were analyzed by immunoblot using an anti-ubiquitin antibody. AWP1-Flag recovered after the pull-down assay was visualized by CBB staining (lower panels). Inputs, 10% of the poly-ubiquitin chains (lane 1) and 2.5% of the cell lysates (lane 5) used for the assay. Pex5 is ubiquitinated at a highly conserved cysteine residue and ubiquitination is a prerequisite for Pex5 shuttling back to the cytosol from the peroxisome 34, 35. However, the molecular mechanism underlying the export of ubiquitinated Pex5 remains undefined. To begin to address this issue, we set out to identify the cys-ubiquitinated form of Pex5 in cells by exploiting the fact that the thioester cys-Ub linkage is highly susceptible to reducing agents such as DTT 34. As previously reported 36, cytosolic (S) and organelle (P) fractions from HeLa cells were subjected to SDS–PAGE in the absence and presence of DTT. Immunoblot analysis using an anti-Pex5 antibody revealed a lower mobility (slower migrating) protein band in addition to unmodified Pex5 in the absence of DTT in the post-nuclear supernatant (PNS), S and P fractions (Figure 6A, upper panel, lanes 1–3, solid and open arrowheads, respectively). In the presence of DTT, the level of the slower migrating form of Pex5 was markedly reduced in the P fraction and partly reduced in the PNS fraction (lanes 4 and 6), but unaffected in the S fraction (lane 5). In Pex5 knock-down cells, which were treated with an siRNA targeting Pex5, the slower migrating band as well as unmodified Pex5 were barely detectable (data not shown). These results indicated that the slower migrating band represents a modified form(s) of Pex5. Furthermore, there appeared to be two types of modified Pex5: a DTT-sensitive form, which is most likely the cys-ubiquitinated form that is associated with peroxisomes in rat liver 45 and rat hepatoma Fao cells 36, and a DTT-insensitive form, perhaps corresponding to a lysine-linked ubiquitinated or other form that localizes predominantly to the cytosol. To verify that the DTT-sensitive form of Pex5 was in fact the ubiquitinated form of the protein, the RING peroxin Pex10, which has been implicated in the ubiquitination of Pex5 39, 40, was inactivated by RNA interference (RNAi) and the effects on Pex5 modification were examined. In PEX10 knock-down HeLa cells, the DTT-sensitive form of Pex5 was no longer detectable in the P fraction (Figure 6A, lower panel, lanes 3 and 6), while the DTT-insensitive form remained discernible in the S and PNS fractions (lanes 1, 2, 4 and 5). These results indicated that the DTT-sensitive form of Pex5 is the cys-linked ubiquitinated form, and that ubiquitination is likely catalyzed by the RING–finger peroxin complex. AWP1 binds to ubiquitinated Pex5. A) PNS fractions from HeLa cells (upper panels) and PEX10 knock-down HeLa cells were separated into cytosol (S) and organelle (P) fractions. An aliquot each of the PNS and S fractions and four aliquots of the P fraction were analyzed by SDS–PAGE in the absence (lanes 1–3) and presence (lanes 4–6) of DTT. Pex5 and the peroxisomal membrane protein marker Pex13 were detected with their respective antibodies. Open and solid arrowheads indicate unmodified Pex5 and ubiquitinated Pex5, respectively. B) HeLa cells were solubilized and incubated with recombinant GST (lane 2), GST-AWP1-Wt (lane 3) and GST-AWP1-C30S (lane 4). Bound fractions were analyzed by SDS–PAGE in the absence (upper panel) and presence (lower panel) of DTT. Pex5 was detected with an anti-Pex5 antibody. GST and GST-AWP1 recovered after the pull-down assay were visualized by CBB (lower panels). Input, 1% of the cell lysate used for the assay. C, Upper panels, an in vitro Pex5 import assay was performed with wild-type 35S-Pex5 (Wt) and the C11A and C11K mutants of Pex5 in the presence of ATP using post-heavy mitochondrial supernatant fraction from rat liver. After incubation, reaction mixtures (Total, T) were treated with 20 mm NEM and separated into cytosol (S) and organelle (P) fractions by ultracentrifugation. Equal aliquots of these fractions were analyzed by SDS–PAGE in the absence (−, upper panel) or presence (+, lower panel) of DTT, as in Figure 1A. Open and solid arrowheads indicate unmodified (Pex5) and ubiquitinated Pex5 (Ub-Pex5), respectively. Lower panel, import reactions of wild-type 35S-Pex5p (lanes 1–4) and the C11K mutant (lanes 5–8) were performed in the presence (lanes 3, 4, 7 and 8) or absence (lanes 1, 2, 5 and 6) of GST-Ub. After incubation, reaction mixtures were fractionated and analyzed by SDS–PAGE in the absence of DTT. Shaded, solid and open arrowheads indicate GST-Ub-Pex5p, Ub-Pex5p, and unmodified, non-ubiquitinated Pex5p, respectively. D) Organelle fractions with imported wild-type 35S-Pex5 (Wt, upper panel) or the C11A (middle panel) and C11K (lower panel) mutants were solubilized and incubated with anti-Flag IgG-agarose beads conjugated with recombinant AWP1-Flag-Wt (Wt) and AWP1-Flag-C30S (C30S), or with agarose beads alone (FL beads). Bound fractions were analyzed by SDS–PAGE in the absence (lanes 1 and 3–5) or presence (lanes 2 and 6–8) of DTT as in C. Open and solid arrowheads indicate unmodified (Pex5) and ubiquitinated Pex5 (Ub-Pex5), respectively. Input, 2.5% of the lysates used for pull-down assays. The ability of AWP1 to interact with ubiquitinated Pex5 was investigated by the glutathione S-transferase (GST)-pull-down assay. HeLa cell extracts were incubated with GST alone, GST-AWP1-Wt or GST-AWP1-C30S. Bound proteins were analyzed by SDS–PAGE in the absence and presence of DTT. DTT-sensitive ubiquitinated Pex5 and unmodified Pex5 specifically bound to GST-AWP1-Wt (Figure 6B, lanes 2 and 3), while only a very low level of unmodified Pex5, but not ubiquitinated Pex5, bound to GST-AWP1-C30S (lane 4). Similar results were obtained using ubiquitinated Pex5 generated in vitro (Figure 6C,D). In an in vitro Pex5 import assay, in the presence of ATP, imported wild-type 35S-Pex5, but not the imported C11A mutant in the peroxisome, was clearly evident as a DTT-sensitive, slower migrating form as well as an unmodified form (Figure 6C, upper panels, lanes 1–6), indicating that Pex5 is ubiquitinated at cysteine 11 via a thioester bond. Similar findings were reported for Pex5 in mammals 39, 40 and yeast 39, 40. In support of the Cys11-ubiquitination of wild-type Pex5 in peroxisomes, similar import assays using 35S-Pex5-C11K resulted in the generation of a DTT-resistant, isopeptide bond-linked ubiquitinated form of Pex5 in the organelle fraction (Figure 6C, upper panels, lanes 7–9) 37. Wild-type 35S-Pex5 and 35S-Pex5-C11K were also ubiquitinated with a GST–Ub conjugate when imported to peroxisomes (Figure 6C, lower panel, lanes 4 and 8), confirming that Pex5p is ubiquitinated in the peroxisome. The 35S-Pex5-imported organelle fractions were solubilized and subjected to a pull-down assay using anti-Flag IgG-agarose conjugated to recombinant AWP1-FL-Wt or its C30S mutant, as described in Figure 5C. The DTT-sensitive ubiquitinated form of wild-type Pex5 bound more efficiently to AWP1-FL-Wt than the DTT-resistant ubiquitinated Pex5-C11K (Figure 6D, upper and bottom panels, lanes 4 and 7, solid arrowheads), whereas unmodified wild-type Pex5 and the C11K and C11A mutants were recovered with both AWP1-FL-Wt and AWP1-FL-C30S (Figure 6D, upper, middle and bottom panels, lanes 4 and 7, open arrowheads). These results strongly suggested that AWP1 preferentially associates with the cys-ubiquitinated form of Pex5 in a manner dependent on its A20 zinc-finger domain. AWP1 also appears to interact with unmodified Pex5 at a lower, basal level. To determine whether the findings from the in vitro assays regarding the potential role of AWP1 in Pex5 export were also true in vivo, the effects of AWP1 knock-down by RNAi on PTS1-protein import into peroxisomes was assessed. In HeLa cells transfected with an AWP1 siRNA, AWP1 expression was barely detectable compared to cells transfected with a control siRNA (Figure 7A, upper middle panel), indicating the efficient knock-down of AWP1 expression. The levels of peroxisomal membrane proteins such as Pex14 and Pex13 were not altered by AWP1 knock-down (Figure 7A, upper middle panel). In control siRNA-treated cells, enhanced green fluorescent protein (EGFP) conjugated to the SKL tripeptide (EGFP-SKL), a model PTS1 cargo, was discernible as a punctate fluorescent pattern indicative of peroxisomal localization (Figure 7A, upper left panel, a). In contrast, EGFP-SKL import into peroxisomes was severely affected in cells transfected with AWP1 siRNA (Figure 7A, upper left panel, b). Moreover, the levels of acyl Co-A oxidase AOx-B, which is generated in vivo by intraperoxisomal conversion of the 75-kDa PTS1-containing protein AOx-A 16, were reduced approximately by 50% in AWP1 siRNA-treated cells (Figure 7A, upper middle panel). In contrast to peroxisomal import, mitochondrial transport appeared to be unaffected, based on the localization of an EGFP-tagged mitochondrial matrix-targeting sequence (MTS-EGFP) and the endogenous matrix protein malate dehydrogenase (Figure 7A, lower panels). These results strongly suggested that AWP1 is required for normal PTS1-mediated protein import. Knock-down of AWP1 decrease PTS1-protein import and affects Pex5 stability. A) HeLa cells stably expressing EGFP-SKL (a and b) or MTS-EGFP (c and d) were transfected twice with control siRNA (left panels, a and c) (middle and right panels, lane 1) or AWP1 siRNA (left panels, b and d) (middle and right panels, lane 2). Forty-eight hours after the second siRNA transfection, EGFP-SKL and MTS-EGFP were visualized by fluorescence microscopy. Scale bar, 10 µm. AOx-B, representing a proteolytically converted 52-kDa form of the 75-kDa AOx-A chain 16, MDH, LDH and AWP1 were detected with their respective antibodies. AOx-B levels in control and AWP1 knock-down cells were quantified relative to the the level seen in control cells, which was set as 1 (right panel). Data represent means ± ranges of two independent experiments. B) HeLa cells were transfected with control siRNA (lane 1), AWP1 siRNA (lane 2) and PEX1 siRNA (lane 3) as in A. Left panel, Pex5, Pex14, LDH, AWP1 and Pex1 in cell lysates (corresponding to 2 × 104 cells) were detected by immunoblot using their respective antibodies. Right panel, Pex5 levels were quantified and normalized to LDH levels in the indicated cell types. Data are presented as in A. C) Control or AWP1 knock-down cells (1 × 107 cells) were homogenized and separated into cytosol (S) and organelle (P) fractions. Equal amounts of each fraction were assessed for Pex5, Pex14, LDH and AWP1 levels, as in B (left panel). Pex5 in S and P was quantified and is shown as in A according to P/(S+P) ratio (right panel). Data represent the means ± SD of three independent experiments. **p 0.025. It is noteworthy that in AWP1 siRNA-treated cells, Pex5 levels were decreased approximately by 50% compared to control siRNA-treated cells (Figure 7B, lanes 1 and 2). Pex5 instability was also noted in human fibroblasts from patients deficient in Pex1, Pex6 and Pex26 54, all of which are required for Pex5 export. Knock-down of Pex1 by RNAi resulted in a reduction in Pex5 to approximately 30% of that seen in control cells (Figure 7B, lane 3), which strongly suggested that Pex5 export is tightly linked to the functions of AWP1 and the AAA ATPase peroxins. Pex5 protein may be unstable and more readily degraded in cells with impaired Pex5 export. When we examined the distribution of Pex5 in control and AWP1 knock-down cells, the level of Pex5 in the cytosol (S) was decreased while Pex5 levels in the organelle fraction (P) were unaffected compared to control siRNA-treated cells, resulting in an increase in the level of Pex5 in the P fraction relative to total Pex5 (P + S) (Figure 7C). This phenotype is similar to that of the Pichia pastoris pex4 mutant 55, providing further evidence that Pex5 export and stability are affected in AWP1-knock-down cells. These results demonstrate that AWP1 is an intrinsic factor that regulates Pex5 export through interactions with Pex6 and mono-ubiquitinated Pex5. In the process of PTS1-protein import into peroxisomes, Pex5 is involved in multiple events, including cargo recognition, targeting to peroxisomes, translocation across the peroxisomal membrane, cargo unloading, ubiquitination, and export back to the cytosol. Export and ubiquitination of Pex5 are ATP-dependent steps 30, 33, 56 and are mediated by several peroxins, including Pex1, Pex6 and in yeast, Pex4 30, 32. Pex4 is an E2 enzyme and is believed to be required for ubiquitination of a cysteine residue in the N-terminal region of Pex5 35. Recently, a member of the UbcH5 family was identified as a potential mammalian ortholog of Pex4 37. Ubiquitination of Pex5 is most likely a signal for Pex5 export, which is mediated by the AAA ATPases Pex1 and Pex6. Pex12 has recently been identified as a putative Pex5 E3 ligase that facilitates the Pex4-mediated ubiquitination of Pex5 in S. cerevisiae40. However, several issues remain unresolved, including how mono-ubiquitinated Pex5 is recognized by the export machinery and how Pex5 is de-ubiquitinated, presumably in the cytosol, for the next round of protein import. Of note with regard to the latter, Ubp15 was very recently identified as a yeast Ub hydrolase for Ub-modified Pex5 57. We previously showed that Pex5 was exported from peroxisomes in a manner dependent on the presence of a cytosolic fraction 30, which strongly suggested the presence of unidentified cytosolic factors that mediate Pex5 export. In the current work, Pex5 export-enhancing factors were identified from rat liver cytosol using chromatographic separation and an in vitro Pex5 export assay. AWP1 was identified as a putative cytosolic Pex5 export factor and its role in Pex5 export was demonstrated based on several lines of evidence. First, Pex5 export was stimulated in vitro by the addition of bacterially expressed and purified AWP1, demonstrating that AWP1 and peroxisome-associated factors, including Pex1, Pex6 and Pex26, are sufficient for Pex5 export (Figure 3). However, given that the in vitro Pex5 export assay system most likely lacks several factors involved in the ubiquitination of Pex5 such as an E1 enzyme and Ub, this result suggested that Pex5 is ubiquitinated prior to the export step. Moreover, the AWP1-C30S variant, which harbored a single point mutation in the A20-type zinc-finger domain, failed to stimulate Pex5 export, excluding the possibility that the Pex5 export-enhancing activity identified in rat cytosol was due to a contaminating protein that co-purified with AWP1 from E. coli. Secondly, AWP1 interacted with the Pex6 AAA ATPase, which is essential for Pex5 export (Figure 4), strongly supporting the idea that AWP1 is a novel cofactor of Pex6. AWP1 contains two zinc-finger domains, an A20 type and an AN1 type 41-43. Mutational analysis demonstrated that the two zinc-finger domains play a pivotal role in the interaction with Pex6, with the A20-type zinc-finger domain perhaps playing a more critical role (Figure 4C). This was supported by the finding that AWP1-C30S failed to enhance Pex5 export (Figure 3). These findings strongly suggest that the interaction between AWP1 and Pex6 is specific and physiologically relevant. AWP1 may stimulate Pex5 export via an interaction with Pex6, wherein AWP1 and Pex1 bind Pex6 on Pex26 in a mutually exclusive manner. Similar to previous studies, we showed that the A20-type zinc-finger domain of AWP1 bound to poly-ubiquitinated proteins (Figure 5A,C) 43, 49 and mono-Ub (Figure 5B,C), indicating that AWP1 is a novel Pex5 export factor that recognizes ubiquitinated proteins. Pex5 in cellular fractions was present as DTT-sensitive and DTT-insensitive forms, representing cys-linked ubiquitinated and lysine-ε-amino-linked ubiquitinated Pex5, respectively (Figure 6A). On the basis of a very recent report identifying a DTT-sensitive, mono-ubiquitinated form of Pex5-Cys11 in Fao cells 36, it seems likely that in HeLa cells, Pex5 is modified at Cys11 with mono-ubiquitiin as well. The two forms of modified Pex5 were also distinguishable by subcellular distribution as peroxisome-associated cys-ubiquitinated Pex5 and cytosolic DTT-insensitive Pex5 (Figure 6A), despite the fact that they were indistinguishable based on migration on SDS–PAGE gels. Furthermore, in PEX10 knock-down cells, the DTT-sensitive cys-ubiquitinated form of Pex5 was absent (Figure 6A), which suggests that ubiquitination is catalyzed by the RING peroxin complex E3 ligase, as has been previously suggested 39, 40. The DTT-insensitive form of Pex5 likely represents lysine-linked ubiquitinated Pex5 generated through the action of another E3 ligase. We were successful in generating cys-ubiquitinated Pex5 in a cell-free system, which allowed us to verify the interaction between AWP1 and cys-ubiquitinated Pex5 (Figure 6C). In an in vitro GST pull-down assay, AWP1 selectively bound to ubiquitinated Pex5 compared to unmodified Pex5 (Figure 6B,D). Point mutation of the critical Cys residue in the A20-type zinc-finger domain of AWP1 abolished this interaction, and significantly inhibited the interaction of AWP1 with unmodified Pex5. It is noteworthy that Pex5 has been shown to function as a tetramer 58, 59, a dimer 21 or a monomer 60, 61. Thus, it is possible that most of the unmodified Pex5 bound to AWP1 (either GST-fused or Flag-tagged AWP1) co-precipitated with ubiquitinated Pex5. Alternatively, it may represent Pex5 bound directly to AWP1. Moreover, point mutation of the critical A20-type zinc-finger domain Cys residue in AWP1 and mutation of the ubiquitination site of Pex5 did not completely abolish the AWP1–Pex5 interaction (Figures 6B,D), suggesting that AWP1 can interact, albeit weakly, with Pex5 in a ubiquitination-independent manner. Thus, AWP1 recognizes and exports ubiquitinated Pex5 in concert with the Pex1–Pex6 complex (Figure 8). With regard to the low recovery of ubiquitinated Pex5 in the GST pull-down assay (Figure 6B,D), one possibility is that the complex of ubiquitinated Pex5 and AWP1 is less accessible to exogenously added AWP1, and is therefore not readily precipitated in a pull-down assay. AWP1 was not detected in anti-Pex5 immunoprecipitates from organelle fractions, presumably because of the very low level of ubiquitinated Pex5 present (data not shown). A model for the Pex5 import and export reaction. During matrix protein import, Pex5 is initially targeted to an 800-kDa complex containing Pex14 30. Pex5 then translocates to a 500-kDa complex containing RING peroxins, where ubiquitination of Pex5 likely takes place. In the terminal step, Pex1, Pex6 and AWP1 catalyze the export of Pex5. The numbers designate peroxins. In the 800-kDa complex, 14n designates the Pex14 oligomer. In HeLa cells depleted of AWP1 by RNAi, PTS1-protein import was severely affected (Figure 7A), clearly demonstrating that AWP1 plays an important role in PTS1-protein import. Furthermore, in AWP1 knock-down cells, the levels of Pex5 protein were decreased (Figure 7B), suggesting a functional interaction between AWP1 and Pex5. In several yeast P. pastoris pex mutants, including pex4, pex1 and pex6, which are all defective at a later step of protein import, Pex5 and Pex20 are degraded in the proteasome by the so-called RADAR pathway 62. Pex5 is likewise destabilized in cells that are deficient in human Pex1 54, human and plant Pex6 54, 63, human Pex26 54 and Hansenula polymorpha Pex4 64, suggesting that the RADAR pathway is also involved in the regulation of Pex5 stability in other species. In good agreement with these previous reports, Pex5 levels were significantly reduced in Pex1 knock-down HeLa cells (Figure 7B). Such a phenotype of Pex5 destabilization in AWP1-depleted cells resembles that of the above-mentioned cell mutants impaired in Pex5 recycling, suggesting that AWP1 functions in a manner tightly linked to these peroxins involved in Pex5 recycling. Incidentally, AWP1 depletion resulted in an increase of Pex5 in organelles relative to the cytosol, and this increase occurred concomitantly with the destabilization of Pex5 (Figure 7C), which supports the idea that AWP1 is required for Pex5 export. Thus, AWP1 is a novel factor essential for Pex5 export and the regulation of PTS1-protein import. AWP1 was first identified as a protein associated with the serine/threonine kinase PRK1, a lipid-activated kinase of the protein kinase C superfamily 41. AWP1 also negatively regulates NF-κB transcription activity 42, 43, raising the possibility that PTS1-protein import may be regulated by PRK1-mediated signals, and that there is some level of crosstalk between PTS1-protein import and NF-κB signaling pathways. Given the finding that there were at least two distinct cytosolic factors that enhanced Pex5 export (Figure 1), it is likely that multiple factors may be involved in the concerted regulation of Pex5 export and PTS1 import. Defining these additional factors will shed new light on the mechanisms of regulation of peroxisomal protein import in response to cellular signals. In mammals, five A20-type zinc-finger-domain-containing proteins have been identified: AWP1, A20, Cezanne, Rabex-5, and AWP1-related protein ZNF216 49. A20, Cezanne, Rabex-5 or ZNF216 could serve as a Pex5 export factor that may be functionally redundant to AWP1. Indeed, ZNF216 exhibits Pex5 export-enhancing activity similar to AWP1 (data not shown). Furthermore, as the A20-type zinc-finger domain was critical for the interaction of AWP1 with Pex6 (Figure 4), it is likely that these four proteins (A20, Cezanne, Rabex-5 and ZNF216) likewise interact with Pex6. A20 and Cezanne are de-ubiquitinating enzymes 65 and A20 and Rabex-5 exhibit E3 ligase activity 66, 67, and both activities could play a role in the Pex5-ubiquitination cycle. Further studies are needed to address the identity and role of additional Pex5 export proteins in other species. It will also be interesting to determine when the function of AWP1 in Pex5 recycling emerged from an evolutionary perspective. To date, homologs of AWP1 in yeast, fungi and plant have yet to be reported. The successful isolation and identification of AWP1 as a Pex5 export factor using a combination of chromatographic protein separation and in vitro Pex5 export assay provide a useful new strategy for screening factors involved in peroxisome biogenesis. The identification of additional factors should help determine the precise mechanism(s) of protein translocation across the peroxisomal membrane. We used rabbit antibodies to Pex14 22, Pex13 68, AOx 69, Flag (Sigma), hemagglutinin A (HA) 21, malate dehydrogenase 30, and affinity-purified IgG to Pex5 21; goat antibodies to LDH (Rockland Gilbertsville) and AWP1 (Santa Cruz; used in Figures B and 7); and a monoclonal antibody to ubiquitin (Medical and Biological Laboratories). A rabbit antibody was raised against amino-acid residues 44–144 of human AWP1 and used in the 35S-Pex5 export assay (see Figure 3D) as described below. Wild-type Chinese hamster ovary (CHO), CHO-K1 and HeLa cells were cultured in Ham\'s F12 medium and Dulbecco\'s modified Eagle medium, respectively, both supplemented with 10% fetal calf serum in 5% CO2 and 95% air 69. A cDNA encoding N-terminally His6- and Flag-tagged or N-terminally Myc3-tagged (used only in Figure 3D) Chinese hamster Pex5L, the longer isoform, was transcribed and translated using a TNT Quick Coupled transcription/translation system (Promega) with 35S-methionine and 35S-cysteine (Perkin-Elmer Japan) 30. E. coli XL1-Blue cells transformed with pGEX6p-1 (GE Healthcare Bioscience) encoding wild-type mouse AWP1-Wt or -C30S mutant fused to GST (GST-AWP1-Wt and GST-AWP1-C30S, respectively) were cultured overnight at 37°C. Each culture was diluted with 100 mL of yeast extract-trypton medium. After an additional 90 min of culture at 37°C, cells were grown at 37°C for 5 h in the presence of 0.2 mm isopropyl-β-D-thiogalactopyranoside (Nacalai Tesque). Cells were harvested, resuspended in 15 mL of suspension buffer containing 20 mm HEPES-KOH, pH 7.4, 0.3 m KCl, 1 mm DTT, 5% glycerol and 1 mm phenylmethysulfonyl fluoride, sonicated, and then centrifuged. The resulting supernatant was mixed with 50 µL of gluthathione-Sepharose beads (GE Healthcare Bioscience) and incubated at 4°C for 2 h. After thorough washing, beads were incubated at 4°C for 1 h with elution buffer containing 20 mm HEPES-KOH, pH 7.4/0.15 m KCl/0.2 m sucrose/1 mm DTT/0.2 mm ATP. Recombinant AWP1 proteins were then eluted from the beads by cleavage of GST with PreScission protease (GE Healthcare Biosciences) in 50 µL of elution buffer without ATP. AWP1-FL-Wt and AWP1-FL-C30S were likewise prepared from their respective GST-fusion proteins. AWP1(44-144) was purified as described 37 and used to generate rabbit antibody. For 35S-Pex5 import into peroxisomes, a heavy mitochondrial supernatant fraction from rat liver was incubated at 26°C for 30 min with 35S-Pex5 in the presence of 3 mm MgCl2 and 50 mm KCl. The import reaction mixture was centrifuged at 18 800 ×g for 15 min. The 35S-Pex5-imported organelle fraction was washed with homogenizing buffer containing 5 mm HEPES-KOH, pH 7.4/0.25 m sucrose/0.1% ethanol/protease inhibitor cocktail (2 µg/mL aprotinin, 25 µg/mL antipain and 25 µg/mL leupeptin) and then resuspended in 2 mL of homogenizing buffer. After centrifugation at 700 ×g for 5 min, the supernatant was divided into 25-µL aliquots, which were frozen in liquid nitrogen and stored at −80°C. For the Pex5 export reaction, 35S-Pex5-imported organelle fractions were mixed with test protein samples in export buffer containing 5 mm HEPES-KOH, pH 7.4/0.2 m sucrose/0.1 m KCl/1 mm DTT and 8.25 µL of an ATP-regenerating system: 30 mm MgCl2/0.1 m creatine phosphate/500 µg/mL creatine kinase, 20 mm ATP, 10% bovine serum albumin and 50 µg/mL recombinant Pex5, and adjusted to 82.5 µL with export buffer or rat liver cytosol fraction. To verify the effect of the anti-AWP1 antibody on 35S-Pex5 export, rabbit antiserum or preimmune serum (10 µL) was added. The reaction mixture was incubated at 26°C for 30 min and then centrifuged at 20 000 ×g for 30 min at 4°C. Supernatant fractions were analyzed by SDS–PAGE. Exported 35S-Pex5 was detected and quantified using a Fujix FLA-5000 Autoimaging analyzer (Fuji Film). Cytosolic proteins from four rat livers in approximately 200 mL were precipitated with 30% (NH4)2SO4, resuspended in 20 mm Tris–HCl, pH 7.0, and desalted using Econo Pac 10 DG column (BioRad) equilibrated with buffer A (20 mm Tris–HCl, pH 7.0, and 1 mm DTT containing 0.15 m KCl). The sample was applied to a 4xHiTrap Q column (GE Healthcare Biosciences) equilibrated with buffer A plus 0.15 m KCl. The flow-through fraction was then applied to a 3xHiTrap SP column (GE Healthcare Biosciences) equilibrated with buffer A plus 0.15 m KCl. Proteins were eluted with a 10-mL linear gradient of 0.15–0.6 M KCl in buffer A and collected in 10 tubes. Proteins in fraction 1 were subjected to ultracentrifugation at 65 000 r.p.m. (370,000 ×g) for 90 min at 4°C in a 5–30% (wt/wt) sucrose density gradient in a Beckman NVT65.2 near-vertical rotor. The gradient was fractionated into 10 500-µL fractions from the base of the gradient. From each fractionation step, equal aliquots were adjusted to 0.1 M KCl and assessed for Pex5 export activity. Fractionated proteins were precipitated with trichloroacetic acid and analyzed by SDS–PAGE and silver staining. A protein band of approximately 40 kDa was subjected to in-gel digestion with trypsin and analyzed by mass spectrometry using an LCQ-Deca IT mass spectrometer (Finnigan). CHO-K1 cells expressing FL-AWP1 alone or in combination with Pex6-HA and Pex1-HA (see below) were lysed in lysis buffer (20 mm HEPES-KOH, pH 7.4/0.1 m KCl/0.5% Nonidet P-40/1 mm DTT/10% glycerol/protease inhibitor cocktail). After centrifugation, cell lysates were incubated with anti-Flag agarose beads (Sigma) at 4°C for 2 h. After thorough washing, immunoprecipitates were subjected to SDS–PAGE and immunoblot analysis. Recombinant AWP1-Wt and AWP1-C30S were incubated for 2 h at 4°C with ubiquitin- or SUMO-conjugated agarose beads (Boston Biochem) in 20 mm HEPES-KOH, pH 7.4/0.15 m KCl/0.2% Triton-X-100/1 mm DTT/5% glycerol/0.5% bovine serum albumin. After thorough washing, proteins bound to beads were analyzed by SDS–PAGE and immunoblot using the indicated antibodies. HeLa cells were lysed in lysis buffer (20 mm HEPES-KOH, pH 7.4/0.1 m KCl/0.5% Nonidet P-40/10% glycerol/5 mm NEM/protease inhibitor cocktail) and then cell lysates (corresponding to 1 × 107 cells) were incubated at 4°C for 2 h with 5 µg each of GST-AWP1-Wt, GST-AWP1-C30S or GST alone. After thorough washing, bound proteins were analyzed by SDS–PAGE in the absence or presence of DTT followed by immunoblot analysis. Subcellular fractionation of HeLa cells was performed as described for CHO cells 30. For the binding assay using ubiquitinated Pex5, wild-type 35S-Pex5 and the two mutants harboring C11A or C11K point mutations were imported into peroxisomes in the presence of ATP and an ATP-regenerating system, as described above. Import reaction mixtures (100 µL each) were incubated with 20 mm N-NEM for 10 min at 4°C and then centrifuged at 18 800 ×g for 15 min. Organelle fractions were washed with homogenizing buffer and solubilized in 1 mL of immunoprecipitation buffer containing 20 mm HEPES-KOH, pH 7.4/0.15 m NaCl/0.1% SDS/1% Nonidet P-40/0.1% bovine serum albumin/5 mm NEM/protease inhibitor cocktail. After insoluble materials were removed by centrifugation, supernatants were incubated for 2 h at 4°C with 15 µL of anti-Flag IgG-agarose beads conjugated with approximately 4 µg each of recombinant AWP1-Flag-Wt and AWP1-Flag-C30S. After thorough washing, proteins bound to the anti-Flag IgG-beads were analyzed by SDS–PAGE in the absence or presence of DTT and then detected using a Fujix FLA-5000 Autoimaging analyzer. The pull-down assay using AWP1-Flag was performed using 1 µg of K48-linked poly-ubiquitin chains (Ub1-7, Boston Biochem) and HeLa cell-lysates (corresponding to 5 × 106 cells), prepared as described above. We used human PEX1-HA, PEX6-HA, FL-PEX267, as well as PEX6-HA variants harboring point mutations in the Walker motifs [A1(K476E), A2(K750E), B2(D803N)], and both A1 and A2 52. To construct mouse Flag-AWP1, a BamHI-NotI fragment of a PCR product generated using mouse brain cDNA as the template and primers 1 and 3 (Table 1) was inserted into the BamHI-NotI sites of pCMV-Flag7. To construct GST-AW P1, a PCR product generated with primers 2 and 3 was likewise inserted into pGEX6p-1 (GE Healthcare Bioscience). To construct GST-AWP1-Flag, an EcoRI-XhoI fragment of a PCR product generated using primers 8 and 9 was inserted into the EcoRI-XhoI sites of pcDNAZeo/C-Flag (Mukai and Fujiki, unpublished) to generate pcDNZeo/AWP1-Flag. Using this construct as a template, a BamHI-NotI fragment of a PCR product generated using primers 2 and 10 was inserted into the BamHI-NotI sites of pGEX6p-1. AWP1-C30S and AWP1-C180S were generated by site-directed mutagenesis 70 using oligonucleotides 4 plus 5 for AWP1-C30S and 6 plus 7 for AWP1-C180S. To generate recombinant AWP1(44-144) for use as an antigen, an EcoRI-SalI fragment of a PCR product generated using primers 11 and 12 was inserted into the EcoRI-SalI sites of pQE30 (Qiagen). Chinese hamster Myc3-PEX5L was constructed by replacing the Hind III-BamH I fragment encoding the His-Flag tag in pcDNAZeo/His-FL-ClPEX5L70 with a double-strand oligonucleotide encoding Myc3-tag, which was generated by annealing the complementing primers 13 and 14. ClPEX5-Wt, ClPEX5-C11A and ClPEX5-C11K in pcDNAZeo vector (Invitrogen) were previously described 36. AGCTTCCACCATGGAGCAAAAGCTCATTTCTGAAGAGGACTTGGAGCAAAAGCTCATTTCTGAAGAGGACTTGGAGCAAAAGCTCATTTCTGAAGAGGACTTGGG GATCCCCAAGTCCTCTTCAGAAATGAGCTTTTGCTCCAAGTCCTCTTCAGAAATGAGCTTTTGCTCCAAGTCCTCTTCAGAAATGAGCTTTTGCTCCATGGTGGA CHO cells were transfected using Lipofectamine reagent (Invitrogen) 71. For knock-down of AWP1 and Pex1, HeLa cells were transfected twice at 24-h intervals with 33 nm of an siRNA targeting human AWP1 (target: UAGUUGUGCCCUAAAGGGCAUUAUC) and human PEX1 (target: AAUGCUUCUUUACAGAUUGCUUUGG) (Stealth/siRNA duplex oligoribonucleotides; Invitrogen) using Lipofectamine 2000 reagent (Invitrogen) 72. A negative control siRNA (Invitrogen) was used as the negative control. A HeLa cell clone stably expressing an shRNA targeting human PEX10 was isolated following transfection of HeLa cells with pSilencer 2.1-U6 puro (Ambion) containing the oligonucleotide 5′-GCTGCAGCTGTACGGTTTCCTTCCTGTCAGAAACCGTACAGCTGCAGCCCTTTTTTGGAAA-3′ inserted into the BamHI and Hind III sites. Selection was carried out using puromycin. EGFP-SKL MTS-EGFP was monitored by fluorescent microscopy using an Axioplan2 fluorescent microscope (Carl Zeiss). We thank M. Matsumoto for mass spectrometric analysis. We also thank T. Umemoto and Y. Sato for the help in the expression and purification of recombinant AWP1(44-144) and M. Nishi for preparing figures, respectively, and the other members of our laboratory for discussion. This work was supported in part by CREST grant (to Y. F.) from the Science and Technology Agency of Japan, Grants-in-Aid for Scientific Research (to Y. F.), and The Global COE Program from The Ministry of Education, Culture, Sports, Science, and Technology of Japan, a grant (to Y. F.) from Japan Foundation for Applied Enzymology. M. N. is a Research Fellow of the Japan Society for the Promotion of Science. Subramani S. Components involved in peroxisome import, biogenesis, proliferation, turnover, and movement. Physiol Rev 1998; 78: 171– 188.Ghaedi K. Lessons from peroxisome-deficient Chinese hamster ovary (CHO) cell mutants. Biochim Biophys Acta - Mol Cell Res 2006; 1763: 1374– 1381. Arabidopsis thaliana--a model organism to study plant peroxisomes. Biochim Biophys Acta – Mol Cell Res 2006; 1763: 1382– 1391.Marten Veenhuis M. Yeast and filamentous fungi as model organisms in microbody research. Biochim Biophys Acta 2006; 1763: 1364– 1373.Fujiki Y. The pathogenic peroxin Pex26p recruits the Pex1p-Pex6p AAA ATPase complexes to peroxisomes. Nat Cell Biol 2003; 5: 454– 460.Kondo N. Identification of a new complementation group of the peroxisome biogenesis disorders and PEX14 as the mutated gene. Hum Mutat 2004; 23: 552– 558.Gould SJ. PEX19 is a predominantly cytosolic chaperone and import receptor for class 1 peroxisomal membrane proteins. J Cell Biol 2004; 164: 57– 67.Fujiki Y. The peroxisomal membrane-protein import receptor Pex3p is directly transported to peroxisomes by a novel Pex19p- and Pex16p-dependent pathway. J Cell Biol 2008; 183: 1275– 1286.Fujiki Y. Peroxisome targeting signal of rat liver acyl-coenzyme A oxidase resides at the carboxy terminus. Mol Cell Biol 1989; 9: 83– 91.Tabak HF. PAS10 is a tetratricopeptide-repeat protein that is essential for the import of most matrix proteins into peroxisomes of Saccharomyces cerevisiae. Proc Natl Acad Sci U S A 1993; 90: 11782– 11786.Veldhoven PPV. Identification and characterization of the putative human peroxisomal C-terminal targeting signal import receptor. J Biol Chem 1995; 270: 7731– 7736.Subramani S. The Pichia pastoris peroxisomal protein PAS8p is the receptor for the C-terminal tripeptide peroxisome targeting signal. EMBO J 1995; 14: 3627– 3634.Kunau W-H. Pex14p, a peroxisomal membrane protein binding both receptors of the two PTS-dependent import pathways. Cell 1997; 89: 83– 92.Fujiki Y. The mammalian peroxin Pex5pL, the longer isoform of the mobile PTS1-transporter, translocates Pex7p-PTS2 protein complex into peroxisomes via its initial docking site, Pex14p. J Biol Chem 2000; 275: 21703– 21714.Fujiki Y. The peroxin Pex14p: cDNA cloning by functional complementation on a Chinese hamster ovary cell mutant, characterization, and functional analysis. J Biol Chem 1999; 274: 12593– 12604.Distel B. Saccharomyces cerevisiae PTS1 receptor Pex5p interacts with the SH3 domain of the peroxisomal membrane protein Pex13p in an unconventional, non-PXXP-related manner. Mol Biol Cell 2000; 11: 3963– 3976.Crane DI. Pex13p is an SH3 protein of the peroxisome membrane and a docking factor for the predominantly cytoplasmic PTS1 receptor. J Cell Biol 1996; 135: 85– 95.Fujiki Y. Restoration by a 35K membrane protein of peroxisome assembly in a peroxisome-deficient mammalian cell mutant. Nature 1991; 350: 77– 81.Gould SJ. PEX12 interacts with PEX5 and PEX10 and acts downstream of receptor docking in peroxisomal matrix protein import. J Cell Biol 1999; 147: 761– 774.Fujiki Y. Molecular anatomy of the peroxin Pex12p: RING finger domain is essential for Pex12p function and interacts with the peroxisome targeting signal type 1-receptor Pex5p and a RING peroxin, Pex10p. J Biol Chem 2000; 275: 25700– 25710.Fujiki Y. Peroxisomal targeting signal receptor Pex5p interacts with cargoes and import machinery components in a spatiotemporally differentiated manner: conserved Pex5p WXXXF/Y motifs are critical for matrix protein import. Mol Cell Biol 2002; 22: 1639– 1655.Fujiki Y. Shuttling mechanism of peroxisome targeting signal type 1 receptor, Pex5: ATP-independent import and ATP-dependent export. Mol Cell Biol 2005; 25: 10822– 10832.Subramani S. The human peroxisomal targeting signal receptor, Pex5p, is translocated into the peroxisomal matrix and recycled to the cytosol. Cell 2001; 105: 187– 196.Erdmann R. Functional role of the AAA peroxins in dislocation of the cycling PTS1 receptor back to the cytosol. Nat Cell Biol 2005; 7: 817– 822.Azevedo JE. Characterization of the peroxisomal cycling receptor, Pex5p, using a cell-free in vitro import system. J Biol Chem 2003; 278: 226– 232.Distel B. A conserved cysteine is essential for Pex4p-dependent ubiquitination of the peroxisomal import receptor Pex5p. J Biol Chem 2007; 282: 22534– 22543.Azevedo JE. Members of the E2D (UbcH5) family mediate the ubiquitination of the conserved cysteine of Pex5p, the peroxisomal import receptor. J Biol Chem 2008; 283: 14190– 14197.Erdmann R. Ubiquitination of the peroxisomal import receptor Pex5p is required for its recycling. J Cell Biol 2007; 177: 197– 204.Distel B. Pex10p functions as an E3 ligase for the Ubc4p-dependent ubiquitination of Pex5p. Biochem Biophys Res Commun 2008; 374: 620– 624.Erdmann R. Pex2 and Pex12 function as protein-ubiquitin ligases in peroxisomal protein import. Mol Cell Biol 2009; 29: 5505– 5516.Teo TS. Cloning and characterization of AWP1, a novel protein that associates with serine/threonine kinase PRK1 in vivo. Gene 2000; 256: 113– 121.Makarov SS. Identification of novel mediators of NF-κB through genome-wide survey of monocyte adherence-induced genes. J Leukoc Biol 2005; 78: 1366– 1377.Prehn JH. Identification of polyubiquitin binding proteins involved in NF-κB signaling using protein arrays. Biochim Biophys Acta 2009; 1794: 1010– 1016.Tyagi AK. A20/AN1 zinc-finger domain-containing proteins in plants and animals represent common elements in stress response. Funct Integr Genomics 2008; 8: 301– 307.Fujiki Y. In vitro import of peroxisome-targeting signal 2 (PTS2) receptor Pex7p into peroxisomes. Biochim Biophys Acta – Mol Cell Res 2009; 1793: 860– 870.Nishimura M. Arabidopsis aberrant peroxisome morphology9 is a peroxin that recruits the PEX1-PEX6 complex to peroxisomes. Plant Cell 2011; 23: 1573– 1587.Karasuyama H. Molecular cloning and characterization of TPP36 and its isoform TPP32, novel substrates of Abl tyrosine kinase. FEBS Lett 2003; 537: 203– 209.Tabak HF. Pex15p of Saccharomyces cerevisiae provides a molecular basis for recruitment of the AAA peroxin Pex6p to peroxisomal membranes. Mol Biol Cell 2003; 14: 2226– 2236.Osumi T. Peroxisome assembly factor-2, a putative ATPase cloned by functional complementation on a peroxisome-deficient mammalian cell mutant. Nat Genet 1995; 11: 395– 401.Fujiki Y. Dynamic and functional assembly of the AAA peroxins, Pex1p and Pex6p, and their membrane receptor Pex26p. J Biol Chem 2006; 281: 27693– 27704.Kunau W-H. Ubiquitination of the peroxisomal targeting signal type 1 receptor, Pex5p, suggests the presence of a quality control mechanism during peroxisomal matrix protein import. J Biol Chem 2005; 280: 1921– 1930.Gould SJ. Multiple PEX genes are required for proper subcellular distribution and stability of Pex5p, the PTS1 receptor: evidence that PTS1 protein import is mediated by a cycling receptor. J Cell Biol 1996; 135: 1763– 1774.Gould SJ. The peroxisome biogenesis factors Pex4p, Pex22p, Pex1p, and Pex6p act in the terminal steps of peroxisomal matrix protein import. Mol Cell Biol 2000; 20: 7516– 7526.Erdmann R. Ubp15p, a ubiquitin hydrolase associated with the peroxisomal export machinery. J Biol Chem 2011; 286: 28223– 28234.Kunau W-H. Recombinant human peroxisomal targeting signal receptor PEX5. Structural basis for interaction of PEX5 with PEX14. J Biol Chem 1999; 274: 5666– 5673.Boekema EJ. The Hansenula polymorpha peroxisomal targeting signal 1 receptor, Pex5p, functions as a tetramer. FEBS Lett 2007; 581: 1758– 1762.Azevedo JE. Pex5p, the peroxisomal cycling receptor, is a monomeric non-globular protein. J Biol Chem 2005; 280: 24404– 24411.Svergun DI. Solution structure of human Pex5·Pex14·PTS1 protein complexes obtained by small angle X-ray scattering. J Biol Chem 2009; 284: 25334– 25342.Subramani S. Dynamics of the peroxisomal import cycle of PpPex20p: ubiquitin-dependent localization and regulation. J Cell Biol 2006; 172: 67– 78.Bartel B. An Arabidopsis indole-3-butyric acid-response mutant defective in PEROXIN6, an apparent ATPase implicated in peroxisomal function. Proc Natl Acad Sci U S A 2004; 101: 1786– 1791.van der Klei IJ. Obstruction of polyubiquitination affects PTS1 peroxisomal matrix protein import. Biochim Biophys Acta 2005; 1745: 176– 186.Dixit VM. De-ubiquitination and ubiquitin ligase domains of A20 downregulate NF-κB signaling. Nature 2004; 430: 694– 699.Bonifacino JS. The Rab5 guanine nucleotide exchange factor Rabex-5 binds ubiquitin (Ub) and functions as a Ub ligase through an atypical Ub-interacting motif and a zinc finger domain. J Biol Chem 2006; 281: 6874– 6883.Fujiki Y. Molecular mechanisms of import of peroxisome-targeting signal type 2 (PTS2) proteins by PTS2 receptor Pex7p and PTS1 receptor Pex5pL. J Biol Chem 2006; 281: 37311– 37320.Fujiki Y. Isolation and characterization of Chinese hamster ovary cell mutants defective in assembly of peroxisomes. J Cell Biol 1990; 110: 651– 660.Fujiki Y. PEX12, the pathogenic gene of group III Zellweger syndrome: cDNA cloning by functional complementation on a CHO cell mutant, patient analysis, and characterization of Pex12p. Mol Cell Biol 1998; 18: 4324– 4336.Ciccarone VC. Advanced transfection with Lipofectamine 2000 reagent: primary neurons, siRNA, and high-throughput applications. Methods 2004; 33: 95– 103. The full text of this article hosted at iucr.org is unavailable due to technical difficulties. Please check your email for instructions on resetting your password. If you do not receive an email within 10 minutes, your email address may not be registered, and you may need to create a new Wiley Online Library account. Can\'t sign in? Forgot your username? Enter your email address below and we will send you your username If the address matches an existing account you will receive an email with instructions to retrieve your username
本文链接: https://www.ebiomall.com/b335-ambion/info-73206.html
免责声明 本文仅代表作者个人观点,与本网无关。其创作性以及文中陈述文字和内容未经本站证实,对本文以及其中全部或者部分内容、文字的真实性、完整性、及时性本站不做任何保证或承诺,请读者仅作参考,并请自行核实相关内容。
版权声明 未经蚂蚁淘授权不得转载、摘编或利用其他方式使用上述作品。已经经本网授权使用作品的,应该授权范围内使用,并注明“来源:蚂蚁淘”。违反上述声明者,本网将追究其相关法律责任。
相关文章
2009-08-04
2011-04-14
2011-10-26
2013-08-24
2014-09-08
2015-01-06
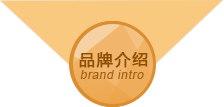
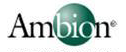
▍
品牌分类
▍
官网分类
▍
品牌问答
暂无品牌问答